Oct. 18, 2013 Research Highlight Biology
Multifunctional molecules aid mutation analysis
Specialized molecular probes that light up on binding to their target allow scientists to quantify DNA samples and detect specific mutations in a single test
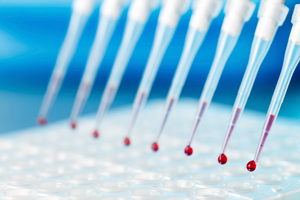
Over the past decade, DNA sequencing technology has become faster and more powerful while also growing more affordable and accessible. As a result, researchers have been able to chart a multitude of genomic variations that could potentially contribute to the development of various cancers as well as a host of hereditary diseases.
Identifying a candidate mutation is only the beginning, however, as independent assays are required to confirm the link between gene and disease. Matthias Harbers and Kengo Usui from the Division of Genomic Technologies at the RIKEN Center for Life Science Technologies have now developed a special class of probes that could accelerate the development of such assays by allowing scientists to simultaneously quantify target DNA sequences while also detecting the presence of mutations of interest1.
Scientists have used variations of a technique called real-time polymerase chain reaction (PCR) to amplify target DNA while monitoring the progress of the amplification. These data are used to determine the original amount of starting genetic material, which can be valuable information in a diagnostic context. Real-time PCR is performed using short stretches of DNA called ‘primers’, which bracket the target sequence of interest and initiate amplification. Harbers and Usui previously worked with a special class of fluorescently labeled molecules called ‘exciton-controlled hybridization-sensitive fluorescent oligonucleotides’ (ECHOs), which feature a pair of dye molecules that quench each other’s fluorescence when in close proximity. When the ECHO binds DNA, the dye molecules become separated as they insert themselves into the double-helix structure, generating a signal that is proportional to the target DNA’s amplification. To efficiently couple this quantitation with the ability to directly detect the presence or absence of mutations, the researchers set out to devise a modified ECHO variant that combines both functions into a single assay.
Dual-purpose probes
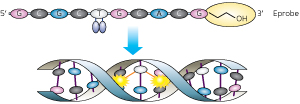
DNA strands that are directly complementary to each other bind more tightly than those that contain mismatches. Scientists can use targeted probes to detect the presence or absence of mutations based on this principle through a technique called melting curve analysis. In contrast, PCR primers generally need to be a perfect match for their target sequence to work reliably. Harbers, Usui and their colleagues therefore opted to modify ECHOs so that they serve as detection probes that act in parallel with a separate set of real-time PCR primers.
The ECHO-based ‘Eprobes’ developed by the research team are designed to bind to potential mutation-containing sites within the region being amplified, allowing amplification detection in real time (Fig. 2). However, Eprobes also contain a chemical group that prevents them from being incorporated into the amplified DNA and instead causes them to dissociate from the target as the sample is heated during the PCR process. Once the PCR has concluded, the Eprobes go back to detecting mutations. “Since their fluorescence activity is still maintained after PCR, we can carry out melting curve analysis as a post-PCR assay in the same tube without additional modification of the reaction mix,” says Usui.
As an initial demonstration, the researchers designed a series of Eprobes to recognize a segment of the gene encoding the epidermal growth factor receptor (EGFR) protein, targeting a mutation associated with cancer risk. The Eprobes performed well in initial experiments, achieving sensitive detection of amplification over the course of the real-time PCR process. Importantly, these Eprobes also proved to be capable of distinguishing between samples that contain only mutant target DNA from those that contain both normal and mutated sequences. Such discrimination is important for many diseases, where a patient’s prognosis can differ depending on whether one or both copies of a chromosome carry a particular mutation. Furthermore, unlike other fluorescent detection tools, Eprobes are completely dependent on DNA binding to generate a signal and are therefore far less likely to produce false positive results that could confound diagnosis.
Monitoring multiple mutations
By combining Eprobes labeled with different fluorescent dyes that exhibit different melting behaviors, scientists could conduct ‘multiplexed’ assays to look at several different mutations or genomic regions simultaneously. To demonstrate this concept, the researchers combined a pair of Eprobes and primer sets to simultaneously investigate both EGFR and KRAS, another gene prone to oncogenic mutations. “At this point, we have two dyes that work well and have also determined that it may be possible to work with Eprobes of three different melting temperatures in one assay,” says Harbers. “This would in theory enable ‘six-plexing’ and could be extended once we have more dyes.” Harbers further notes that the expansion of these multiplexing capabilities will be a key objective in enabling Eprobes to achieve their potential as diagnostic tools.
The research team is currently working with clinicians at Gunma University Hospital to test the sensitivity of these reagents for detecting relatively scarce mutations within the highly heterogeneous context of a tumor. “The frequency of mutation in samples from cancer patients is usually quite low—under 5 per cent—and the detection of such rare mutations is our next challenge,” says Usui. This technology is also undergoing commercial development through K.K. DNAFORM, a RIKEN venture company, and scientists may soon be able to obtain customized Eprobes for a variety of imaging applications. For example, sequence-specific Eprobes could be designed for mapping chromosomal regions of interest, allowing scientists to directly identify clinically relevant chromosomal abnormalities using a microscope. “Any disease for which the outcome or treatment course can be determined by mutation detection could potentially be targeted by our technology,” notes Usui.
References
- 1. Hanami, T., Delobel, D., Kanamori, H., Tanaka, Y., Kimura, Y., Nakasone, A., Soma, T., Hayashizaki, Y., Usui, K. & Harbers, M. Eprobe mediated real-time PCR monitoring and melting curve analysis. PLoS ONE 8, e70942 (2013). doi: 10.1371/journal.pone.0070942
About the Researcher
Matthias Harbers and Kengo Usui
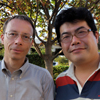
Matthias Harbers was born in Göttingen, Germany, in 1960. He received his PhD from the University of Hamburg in 1989. Harbers worked in Sweden and France before joining RIKEN as a visiting scientist at the Omics Science Center, which was later reorganized to the RIKEN Center for Life Science Technologies. As a member of the FANTOM Project, Harbers has contributed to the development of full-length cDNA cloning and analysis methods (Cap Analysis of Gene Expression (CAGE) technology). His current work focuses on advancing molecular diagnostic methods.
Kengo Usui was born in Kasugai in 1974. After obtaining his PhD from Gifu University in 2003, Usui worked as a researcher for the Japan Science and Technology Agency's CREST program, where he developed self-assembling proteins known as ‘Nanolego’. In 2008, he joined the RIKEN Omics Science Center and has since been conducting research into the development of a rapid genetic diagnostic system. Usui became unit leader of the Genetic Diagnosis Technology Unit at the RIKEN Center for Life Science Technologies in 2013.