Jun. 22, 2018 Feature Highlight Biology
Lighting up the depths of the brain
By using special nanoparticles to convert infrared radiation into light deep inside the brain, neuroscientists can safely explore new areas
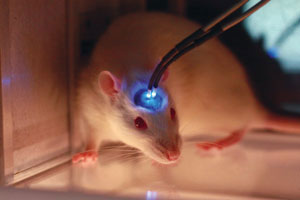
Neuroscience is in the grips of transformation. In the quest to understand the brain’s messenger systems, researchers no longer have to rely on crude electrodes to prod and probe. Instead, they study the brain using highly focused laser beams of visible light that stimulate neural circuits in living, even moving, animals. This technique, known as optogenetics, was developed about a decade ago and has resulted in a huge growth in neuroscience’s output.
However, there is a fundamental problem with using light on brain matter—it cannot travel far before it is absorbed or scattered, which has limited the reach of optogenetics to about half a millimeter into the brain. To overcome this restriction, neuroscientists insert optical fibers (see image), but these fibers sometimes damage surrounding brain tissue. Now, in a study published in Science, Thomas McHugh of the RIKEN Center for Brain Science and his co-workers have found a way around this problem: they are using a near-infrared beam and ‘upconverting’ it to visible light in the relevant brain region by injecting sophisticated nanoparticles1.
Deeper with near-infrared light
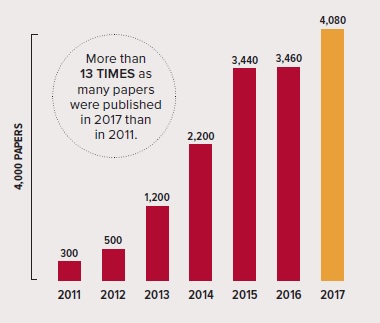
Near-infrared light can travel considerably further into the brain than visible light. But near-infrared light cannot stimulate neurons, so McHugh’s team had to devise a means to convert it into visible light within the brain.
Converting high-energy ultraviolet light into lower energy visible light is fairly simple—scientists have a plethora of fluorescent dyes at their disposal that do this. But going in the opposite direction and changing low-energy infrared light into more energetic visible light is more of a challenge. Two or more particles, or photons, of infrared light need to pool their energies to produce a single photon of visible light. The process requires special particles known as upconversion nanoparticles, so-named because they can convert low-energy photons into higher energy ones.
It was at this point that the expertise and connections of Shuo Chen, a postdoctoral researcher in McHugh’s lab, proved invaluable. “Chen did a PhD in chemistry, and he knew people working on particle synthesis,” says McHugh. “He came to my lab to do optogenetics and said ‘I think there are ways we can improve this tool if we talk to the right chemists.’”
Teaming up on upconversion
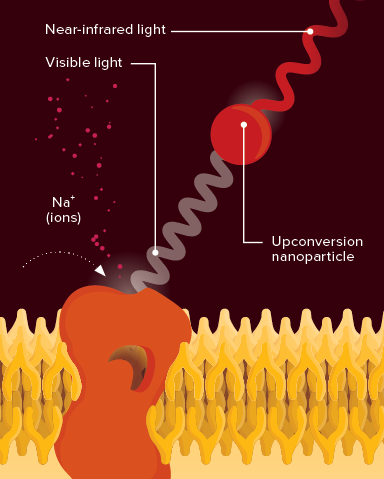
Chen connected McHugh with chemists at the National University of Singapore who specialize in making upconversion nanoparticles. When McHugh’s team injected these nanoparticles close to a brain region of interest, they were able to convert the near-infrared light into visible light, which in turn stimulated neurons. This process has extended the reach of optogenetics to about 4.5 millimeters—roughly a tenfold improvement on the conventional method and about the same depth as that of a mouse brain.
Using this method, the team has already been able to stimulate the release of dopamine, trigger the recall of memories and modify mouse fear responses.
Wolfgang Parak, who co-authored an accompanying article on the paper’s findings in Science, is confident of the importance of overcoming the visible light “dilemma”. Parak, who is from the Center of Hybrid Nanostructures at the University of Hamburg, Germany, and his two co-authors, chose to focus their article on the possible future applications of the method to control neurological issues in human patients, such as those with Parkinson’s disease. Without it, they note that altering brain activity in a “substantial and targeted way” requires a complex tangle of electrodes. Due to this and the size of conventional electrode arrays, they point out that up until now optogenetics has had “severely limited clinical applications”.
Making the method safe
However, there is still much testing to be done. McHugh’s team has already had to address a number of challenges to get their technique to work. “As a biologist, there were two things that raised red flags,” he says. “One was that these nanoparticles use heavy metals to work the magic of upconversion. And when I think of heavy metals, I think of poison!” To get around this problem, the team made the particles biocompatible by encasing them with a silica coating.
The second challenge was ensuring that the near-infrared light did not cook the rodents’ brains. Since infrared radiation is essentially heat, even low levels of absorption can heat a material. Fortunately, simulations performed by the team revealed that blood absorbs a lot of the infrared light, and so a lot of the heat is quickly circulated away.
New clinical and research possibilities
In the next phase, McHugh points to two areas of research that could benefit. The first is the study of the brain stem. Because the brain stem is very deep and contains many highly sensitive nuclei, any damage can severely affect an animal’s health and behavior.
The other area is studying how the brain develops in infant mice. “Researchers who study development have been behind on this optogenetics bandwagon because they can’t use it—they can’t put a fiber in something so small,” McHugh says. “But I think this will work. I’m pretty optimistic: we’re going to try to use it to do pattern simulation in the brains of one- to two-day-old mice.”
As for use in human patients, Parak and his colleagues suggest that the method might eventually be used to “optically control neuronal dysfunctions, such as Parkinson’s disease or even paralysis.”
McHugh is also optimistic, and says that in the near future it might also find application in treating peripheral nerves for chronic pain.
References
- 1. Chen, S., Weitemier, A. Z., Zeng, X., He, L., Wang, X., Tao, Y., Huang, A. J. Y., Hashimotodani, Y., Kano, M., Iwasaki, H. et al. Near-infrared deep brain stimulation via upconversion nanoparticle-mediated optogenetics. Science 359, 679−684 (2018). doi: 10.1126/science.aaq1144
About the Researcher
Thomas McHugh
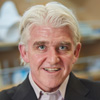
Thomas McHugh majored in molecular and cellular biology at the University of California, Berkeley, before moving to the Massachusetts Institute of Technology (MIT) where he finished a PhD in biology. At MIT, he studied genetics and the physiology of spatial memory with Matt Wilson and Susumu Tonegawa, and continued to study the circuits of hippocampal memory as part of his postdoctoral studies. In 2009, he moved to what is now known as the RIKEN Center for Brain Science to start his own laboratory. His Laboratory for Circuit and Behavioral Physiology at RIKEN takes a multidisciplinary approach to understanding how memories are formed, stored and recalled in the mammalian brain, and how damage from factors such as stress and disease impair these functions.