Nov. 24, 2006 Research Highlight Physics / Astronomy
All the world’s power in your laboratory
Noble gases compress laser pulses to achieve extreme powers
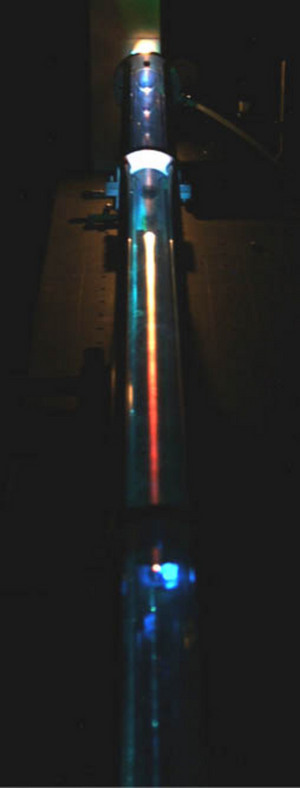
Exciting new physics can be discovered when looking at materials on extremely short timescales or with very high laser powers. However, no laser in itself can produce such short, intense pulses of light. Instead, the laser beams need to be manipulated externally.
For this task, physicists employ the direct relationship between the wavelengths contained in the laser pulse and its duration. Typically, the so-called self-phase modulation effect is used, which is based on a complex interaction of intense light and matter that leads to a broadened laser spectrum necessary for pulse shortening. Argon is one of the ideal candidates for self-phase modulation, as only noble gases are able to withstand the extreme experimental conditions. Other matter would simply be destroyed by the high intensity of the laser beam.
There are different experimental designs to guide light through argon. Common to all approaches is that light is allowed to travel through the gas within a confined space. However, a team from the RIKEN Discovery Research Institute, Wako, with researchers from Tokai University, Hiratsuka, and Brawijaya University, Indonesia, has now uncovered a way to significantly improve on earlier methods. “Light is only confined in one direction perpendicular to the beam, but is free to spread itself in the other,” explains Akira Suda from the RIKEN team. In previous designs such as hollow optical fibers (Fig. 1), light was confined in all directions. However, in the new design, which is rectangular, light is less confined so more spread out allowing significantly higher laser powers across the structure.
The team recently published their new approach in the journal Physical Review Letters1. Solving complicated equations describing the propagation of the laser beam through the gas, the researchers are able to quantify the significant advantage of their approach. “In our design, the peak power a laser pulse can achieve exceeds 140 terawatts, about 100 times the average electric power consumption in the whole world,” says Suda. Of course, all this is restricted to an extremely short period of time. The researchers calculate that the pulse lengths of the laser can be as short as 3 fs—a mere three billionths of one millionth of a second.
The theoretical computations and predictions are very encouraging and as the structure is relatively straightforward to build, the RIKEN team is now attempting to realize the concept experimentally.
References
- 1. Nurhuda, M., Suda, A., Bohman, S., Yamaguchi, S. & Midorikawa, K. Optical pulse compression of ultrashort laser pulses in an argon-filled planar waveguide. Physical Review Letters 97, 153902 (2006). doi: 10.1103/PhysRevLett.97.153902