May 25, 2007 Research Highlight Biology
Coordinating connections between neurons
‘Reverse signaling’ orchestrates synaptic activity
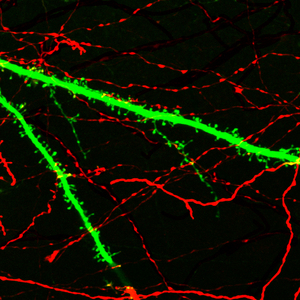
The architecture of the brain is bewilderingly complex. Upwards of one hundred trillion connections between tens of billions of neurons—some individual neurons making thousands of connections to other neurons (Fig. 1)—collectively function to bring about thought, action, emotion, sight and memory. For any of these brain functions to occur, the myriad neuronal connections must be coordinated. Yet, the challenge of coordinating these many connections stems from the physical structure of the synapse.
Neuronal axons—the long ‘arms’ of neurons that carry signals to dendrites—end in terminals that function as exit points for chemical neurotransmitters that bind to receptor molecules expressed on the terminals of dendrites, called spines, which are variously sized and shaped projections from other neurons. Chemical-electrical signals that propagate along axons are ‘delivered’ across a cleft—or synapse—spanning 20–40 nanometers (Fig. 2), to neighboring dendrites that pass along the signals.
Because chemical and molecular activity at pre- and post-synaptic sites is required for successful propagation of signals across synapses, the two synaptic termini must be precisely coordinated. Although signs that regulation of pre-synaptic activity occurs from post-synaptic sites as been reported, the mechanics of such regulation are little understood.
Pinning down the mechanism
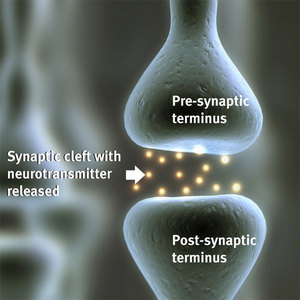
Now, a collaboration of scientists led by Kensuke Futai, Tsutomu Hashikawa, Morgan Sheng and Yasunori Hayashi at the RIKEN-MIT Neuroscience Research Center, Cambridge, US, and the RIKEN Brain Science Institute, Wako, have determined the mechanism by which feedback regulation—or retrograde transmission—coordinates the activity of at least some synapses1.
“Since Ramon Cajal established the synapse hypothesis in [the] 19th century, many people have believed that the major direction of synaptic transmission is the anterograde [or forward] transmission,” remarks Futai on the rationale for the new work. Studies since Cajal’s pioneering work by many scientists found that pre- and post-synaptic structures are tightly coordinated in both size and function, leading some scientists to speculate that feedback, or reverse signaling, by post-synaptic dendrites regulates pre-synpatic activity.
The RIKEN team’s working assumption was that signals from the spines would physically travel ‘in reverse’ across synaptic clefts (Fig. 2) to influence the function and properties of pre-synaptic terminals. Initial experiments centered around three proteins known to connect post-synaptic and pre-synaptic activity: post-synaptic protein 95 (PSD-95), neuroligin and the pre-synaptic protein β-neurexin.
The team first explored the role of PSD-95 in coordinating synaptic activity. By altering the amount of PSD-95 in post-synaptic sites with state-of-the-art molecular biology techniques such as RNA interference and gene delivery with DNA-coated gold particles, and then measuring electrophysiological activity in the synapse, they found that post-synaptic PSD-95 regulates pre-synaptic activity.
They next turned their attention to neuroligin, which is also found in post-synaptic sites. But neuroligin also extends into synaptic clefts where it can interact with proteins on pre-synaptic neurons.
Speculating that neuroligin and β-neurexin—along with PSD-95—could function as a physical bridge linking post- and pre-synaptic neurons, the team measured the electrophysiological activity between neurons after altering the levels of either post-synaptic neuroligin or pre-synaptic β-neurexin. As with PSD-95, changing the amount of either protein altered activity of the synapse.
According to the model developed by Futai and colleagues, post-synaptic PSD-95 and neuroligin play a central role in directly regulating pre-synaptic β-neurexin. But what is ultimately being regulated in the pre-synaptic terminals? The team focused on release of neurotransmitter into synaptic clefts, as β-neurexin had been linked to the molecular apparatus required for neurotransmitter release.
Indeed, by directly measuring the amount of neurotransmitter released from pre-synaptic terminals after altering PSD-95 and neuroligin levels in post-synaptic neurons, and inhibiting the β-neurexin function in pre-synaptic neurons, the team found that all of these manipulations affected neurotransmitter release. A relatively complete picture of the retrograde signaling mechanism was now evident: the PSD-95—neuroligin—β-neurexin complex physically spans synaptic clefts and regulates the release of neurotransmitter.
Importantly, the model posits that retrograde signaling depends on fluctuations in the amount of PSD-95 in post-synaptic terminals, which some studies have shown to be influenced by even simple tasks such as opening one’s eyes. “We hypothesize that neuronal activity such as learning induces localization of PSD-95, and then PSD-95 strengthens the synaptic connection through the neuroligin—β-neurexin interaction,” explains Futai.
Modulating synapses in diseased brain
The work by the RIKEN team may provide new avenues of research on several brain diseases. Retrograde communication may be critical for memory formation and in such diseases as Alzheimer’s or in the development of autism. More generally, the work significantly adds to our understanding of how synapses in general are regulated.
“Beta-amyloid accumulation, which is a major symptom of Alzheimer's disease, causes the loss of PSD-95 from synapse,” says Futai. “It’s perhaps not unexpected that Alzheimer's patients have less PSD-95 since Alzheimer’s patients cannot maintain memories, and because PSD-95 is important for synaptic function.” Yet, increasing the amount of PSD-95 in Alzheimer-diseased neurons to treat memory problems is not very realistic at present, he adds.
Other types of trans-synaptic molecules factors still await intensive study. “I would like to apply [the] same technology we used [in] the present study to other trans-synaptic and retrograde molecules. By doing further studies, I think we will understand better how synapses [memories] are maintained [stored] in the brain,” says Futai. “Regarding further work on PSD-95–neuroligin–β-neurexin, I would also like to know if a relationship exists between these protein-protein interactions and the development of autism.”
References
- 1. Futai, K., Kim, M.J., Hashikawa, T., Scheiffele, P., Sheng, M. & Hayashi, Y. Retrograde modulation of presynaptic release probability through signaling mediated by PSD-95–neuroligin. Nature Neuroscience 10, 186–195 (2007). doi: 10.1038/nn1837
About the Researcher
Yasunori Hayashi and Kensuke Futai
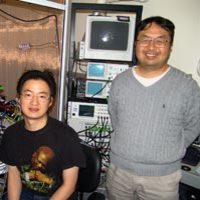
Yasunori Hayashi (picture right, standing) received his MD degree in 1990 and his PhD in Physiology in 1994, both from Kyoto University. He conducted postdoctoral work in AMPA receptor phosphorylation in Tomoyuki Takahashi's laboratory (the University of Tokyo). In 1996 he moved to Cold Spring Harbor Laboratory and conducted research in AMPA receptor trafficking with Roberto Malinow. He is currently a unit leader at the RIKEN Brain Science Institute, as well as an assistant professor at the Picower Institute for Learning and Memory at the Massachusetts Institute of Technology.
Kensuke Futai (picture left, seated) was born in Madison, USA, in 1971. He studied at the Department of Biology in Kyusyu University for his undergraduate and Master’s degrees from 1991 to 1997, before obtaining his PhD at the Department of Neurophysiology at the University of Tokyo in 2001. In the same year, he joined the RIKEN-MIT Neuroscience Research Center, the Picower Center for Learning and Memory (now the Picower Institute for Learning and Memory since 2002) and the Department of Brain and Cognitive Science at the Massachusetts Institute of Technology, as a postdoctoral fellow. Between 2002 and 2005, he held an appointment as a special postdoctoral researcher at the Laboratory for Neural Architecture, in the Advanced Technology Development Group of the RIKEN Brain Science Institute in Japan. His research interests include the investigation of the functional significance of trans-synaptic molecules.