Nov. 30, 2007 Research Highlight Chemistry
Chemical reaction singled out
A reversible reaction cycle is demonstrated for a single molecule sitting on a platinum surface
Chemical reactions are traditionally performed in flasks containing vast numbers of molecules that all undergo the same transformation at more or less the same time. These processes are often studied by monitoring changes in the collective properties of the system, such as concentration, temperature or color.
Although valuable information about how a reaction works can be obtained in this way, it represents only an average of the behavior of all the molecules. To better understand what happens to any given molecule during a chemical reaction, a drastically different approach is required.
With a scanning tunneling microscope (STM), it is possible to ‘see’ individual atoms and molecules sitting on a surface by mapping their electron density. Moreover, the atomically sharp tip of an STM can also be used to manipulate molecules—they can be moved, their shape can be changed, and chemical bonds can be either made or broken.
Chemical reactions induced with STM tips are usually irreversible and not very selective for a particular bond. But now, a team led by Yousoo Kim and Maki Kawai from RIKEN’s Discovery Research Institute in Wako have performed a highly specific and reversible reaction on an isolated molecule. “This is the first time anyone has been able to break and reform a single molecular bond,” comments Kim.
Scratching the surface
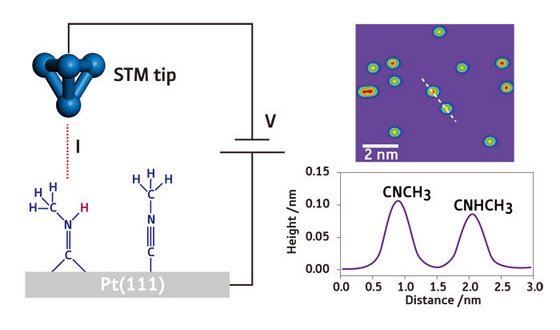
In their study1, reported in the journal Science, Kim and co-workers began by exposing a clean platinum surface to a small number of methylisocyanide (CH3NC) molecules. The molecules adsorb to the surface by forming NC–metal bonds and an STM image revealed that they were located at so-called ‘on-top’ sites, where they bind to just a single platinum atom.
When the sample was treated with hydrogen gas (H2), the platinum surface catalyzed a hydrogenation reaction in which the CH3NC molecules were converted into methylaminocarbyne (CH3NHC) molecules. This process resulted in significant changes to the electronic and geometric structure of the adsorbed molecules, causing them to shift to bridging—rather than ‘on-top’—sites in which they bond to two surface platinum atoms.
A first glance, the STM image taken of the surface after the reaction was not very different from that obtained beforehand; in both cases, molecules simply appeared as bright protrusions. On closer examination, however, it was found that the height profile of these bright spots had decreased after the samples had been treated with H2. This change suggests that a transformation of the surface molecules had occurred, because theoretical models indicated that the CH3 group in CH3NHC should be 0.1 nm closer to the surface than the corresponding group in CH3NC.
In an elegant control experiment (Fig. 1), it was confirmed that this difference in height profile was an intrinsic property of the molecules rather than an artifact of the experiment. Kim and co-workers added extra CH3NC molecules to a sample that had already been treated with H2, and an STM image of this surface—on which both CH3NC and CH3NHC molecules were now present—contained bright protrusions of two different heights, confirming that the two different adsorbed species could be distinguished in this manner.
Taking the pulse
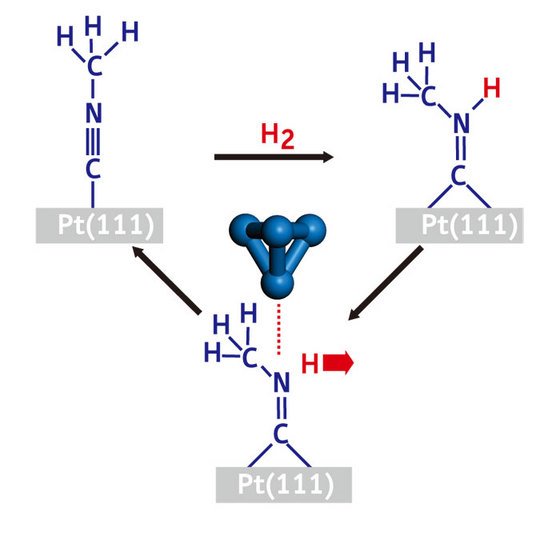
The most significant finding made during this study was that individual CH3NHC molecules could be converted back, one at a time, into CH3NC molecules with the STM tip (Fig. 2). This reverse reaction, in which the N–H bond is broken, was done by precisely positioning the STM tip above the center of a CH3NHC molecule, and injecting tunneling electrons into it by applying a voltage pulse.
Kim and co-workers found that this reverse reaction was sensitive to the magnitude of the voltage pulse. At a constant tunneling current of 1 nA and a pulse duration of 1 second, any voltage less than 2.7 V had no effect on the CH3NHC molecules. However, with pulses ranging from 2.7–3.0 V, the N–H bond of a CH3NHC molecule was selectively cleaved to reform a CH3NC molecule. This change in chemical structure was, as expected, accompanied by a shift in adsorption site as the molecule reverts back to an ‘on-top’ site rather than a bridging one.
At voltages greater than 3.0 V, the CH3NHC molecules were not cleanly converted back into CH3NC molecules, but rather decomposed to give an unidentified product. It is thought that not only the N–H bond breaks at these values, but also C–H bonds in the CH3 group. Breaking of the N–CH3 bond could be ruled out, because the molecular fragments that would be formed in this process were not observed.
Looking to the future
Because the molecule–metal interface plays an important role in the conductance of molecular electronic devices, Kim suggests that being able to control interactions between single molecules and a metal surface may prove useful for such applications. Most studies to date, however, have focused on using sulfur containing compounds known as thiols to link molecules to metal electrodes.
From the standpoint of molecular devices, little experimental and theoretical research has been done on compounds containing this group. As demonstrated in this work, such molecules are appealing because the way in which they interact with a metal surface—such as bonding to one or two platinum atoms—can be reversibly modified without causing any major structural changes.
In addition to the potential of this work for applications in molecular electronics, this study could also offer a greater fundamental understanding of how chemical reactions occur on surfaces at the level of individual molecules.
References
- 1. Katano, S., Kim, Y., Hori, M., Trenary, M. & Kawai, M. Reversible control of hydrogenation of a single molecule. Science 316, 1883–1886 (2007). doi: 10.1126/science.1141410
About the Researcher
Yousoo Kim
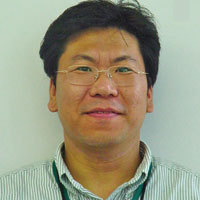
Yousoo Kim was born in South Korea’s capital, Seoul, in 1968. In 1991, he graduated from the Department of Chemistry, Seoul National University, and obtained his masters at the same university in 1993. In 1999, he earned his doctorate in applied chemistry at the University of Tokyo. In the same year, he joined the Surface Chemistry Laboratory at the RIKEN Discovery Research Institute as a research associate, and six months later became a special postdoctoral researcher. In 2002, he was promoted to research scientist. Since 2006, he has been serving as a senior research scientist at the same laboratory. His research focuses on the fundamental studies of electron-molecule interaction at solid surfaces at the single-molecule level.