Jan. 25, 2008 Research Highlight Biology
Nucleus demonstrates its independence
New view of how cells move to form the brain
A RIKEN-led research team has shown that the nuclei of migrating nerve cells in the mouse brain move independently of the centrosomes, the organelles previously thought to pull them along. The work is significant because of the key role nerve cell migration plays in the development of the brain. Any disruption to migration is likely to have an impact on the final position and integration of the cells. This can lead to serious disorders such as lissencephaly or smooth brain syndrome, and has also been implicated in conditions such as epilepsy, schizophrenia and bipolar disease.
Neural migration
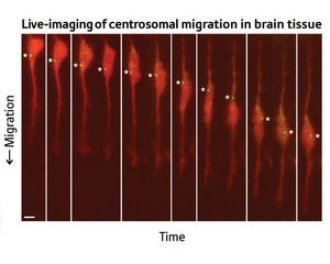
Nerve cells or neurons are generated on the brain’s inner surface and migrate radially outwards to where they end up functioning. Although neural migration is most evident in young animals during brain development, it continues throughout adult life.
Neurons differ from other migratory cells in the long, thin projections extending from their cell bodies known as processes. During migration, the neuron extends such a process in the direction of movement. Then the cell body with all its organelles—the largest of which is the nucleus—moves down that leading process.
In previous studies, researchers observed that the centrosome was nearly always positioned ahead of the nucleus during migration. The centrosome is the main organizing center for the strings of structural proteins called microtubules which form the internal skeleton of the cell. It was therefore suggested that the nucleus was connected by microtubules to the leading process via the centrosome, and was thereby dragged along.
But in a paper published recently in the Proceedings of the National Academy of Sciences1, researchers from Kyoto University and the RIKEN Brain Science Institute in Wako have shown otherwise. In mouse brain slices, using time lapse photography under a confocal microscope, they observed the migratory movement of granule cells in which the centrosomes and nuclei had been stained different colors (Fig. 1). The nuclei exhibited a jumping motion, alternating fast movement where they took on an elongated form, with slow ‘resting phases’ where they returned to a more rounded shape. At times the nuclei moved ahead of the centrosomes.
Microtubular connections
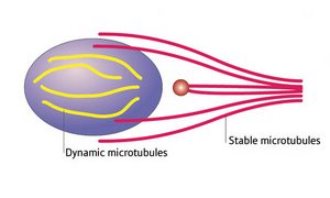
The researchers then stained for different forms of microtubules—the stable form, rich in acetyl groups, and the dynamic form, rich in tyrosine. They found the nucleus was connected to the leading process directly (Fig. 2) by a whisk-like structure composed of stable microtubules—there was no intervening link to the centrosome, which is separately bound to the leading process. In addition, the nucleus was encased in a cage-like structure of dynamic microtubules.
The stable microtubules, in particular, appear to be critical to nuclear movement. Migrating cells were treated with different doses of a compound that is known to disrupt dynamic microtubules at a low level and stable microtubules at a higher level. Only the higher dose, however, halted nuclear movement. Another compound which boosts the formation of stable microtubules also disrupted nuclear movement.
The researchers found that inhibiting LIS1—a gene product which binds with the protein dynein—could stop nuclear movement, but not that of the centrosome. Dynein acts as a motor which walks along microtubules in a rack and pinion motion pulling attached compounds with it. LIS1 binds dynein to the microtubule bundle, and has previously been associated with a neural migration disorder.
Piecing together the whole picture
Pulling all this evidence together, the researchers say it is clear that in mouse granule cells the centrosome and nucleus move independently. And while dynein is an integral part of the nuclear movement process, the centrosome seems to move by some different mechanism.
Project coordinator Mineko Kengaku of the Brain Science Institute suggests it is possible that stable microtubule bundles act as guiding rails connecting the nucleus with the end of the leading process, and down which it is dragged. “Dynein functions like a truck carrying its nucleus cargo along the microtubules.”
She also thinks that the dynamic bundles may simply be a younger form of microtubule, which may well be converted to the stable form at a later time. Dynamic microtubules appear to be generated or prepared by the centrosome, she says. They have been observed radiating from the centrosome like a comet’s tail.
In fact, the role of the centrosome in neuronal migration is the next question the research group hopes to unravel. “We want to uncover the complete mechanism of nuclear and centrosomal movement,” Kengaku says.
References
- 1. Umeshima, H., Hirano, T. & Kengaku, M. Microtubule-based nuclear movement occurs independently of centrosome positioning in migrating neurons. Proceedings of the National Academy of Sciences USA 104, 16182–16187 (2007). doi: 10.1073/pnas.0708047104
About the Researcher
Mineko Kengaku
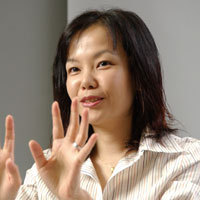
Mineko Kengaku was born in Chigasaki, Japan, in 1966. She graduated from the University of Tokyo in 1989 and in 1995 received her PhD degree at the same institution. She completed a postdoctoral fellowship at Harvard Medical School, and became Assistant Professor at Kyoto University in 1998. She was made laboratory head at RIKEN Brain Science Institute in 2003.
Kengaku studies how neuronal circuits in the brain are formed during development. She has revealed molecules involved in the control of cell shape and positioning during neural circuit formation, primarily in the developing mouse cerebellum. Inappropriate regulation of cell shape and positioning may underlie devastating brain malformation and mental disorders, such as schizophrenia and bipolar disorder. Her research may lead to novel strategies for therapy to treat these diseases.