Feb. 16, 2009 Research Highlight Physics / Astronomy
A penetrating view of viruses
The powerful x-rays from a RIKEN synchrotron can provide high-contrast images of biological specimens
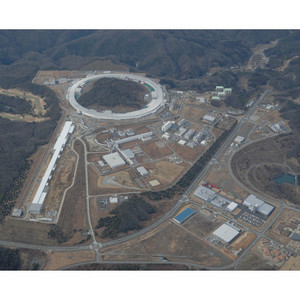
X-ray diffraction is a widely used tool to probe the three-dimensional structures of crystals and large molecules. One of its first successes, in the 1950s, was to reveal the structure of the oxygen-binding proteins hemoglobin and myoglobin, and it has since found many other uses in biology.
More recently, researchers have used x-ray diffraction in a powerful new kind of microscope, which provides high-contrast images of biological cells, viruses or proteins. This so-called ‘x-ray diffraction microscopy’ requires extremely bright, coherent x-rays that are only available at large synchrotron facilities.
Now, Changyong Song at the RIKEN Advanced Science Institute and co-workers have used the synchrotron radiation source at the RIKEN SPring-8 Centre (Fig. 1) in Hyogo prefecture, Japan, to provide the first clear x-ray diffraction images of single, unstained viruses1. The viruses are the smallest objects ever resolved using this technique.
Crystal conundrum
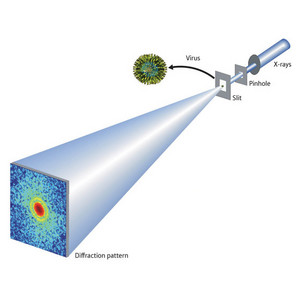
It can be very difficult to crystallize biological samples into a state suitable for traditional x-ray diffraction studies. X-ray diffraction microscopy provides a solution to this problem. It works by measuring the diffraction patterns of non-crystalline samples and then ‘oversampling’ them with a computer algorithm to obtain a sample image at high resolution.
“I previously worked on resonant x-ray scattering, which can resolve complex ground states of many intriguing materials, but this was limited to high-quality crystallized samples,” explains Song, who was previously based at the University of California in Los Angeles. “Later I heard about x-ray diffraction microscopy, which can unravel structures even from a non-crystalline specimen.”
Unfortunately, non-crystalline samples do not amplify the x-ray signal in the same way that crystals do, so the scattered x-rays can be hard to detect. For this reason x-ray diffraction microscopy has so far been limited to imaging samples that are larger than a micrometer in size, or contain heavy elements.
The SPring-8 synchrotron changes all this. Its incredibly powerful x-rays provide very strong diffraction signals, allowing researchers to view much smaller objects.
Viral viewing
Song and co-workers positioned a pinhole aperture to filter x-rays from the synchrotron onto a mouse herpes virus (Fig. 2). The x-rays diffracted by the virus were captured on a CCD camera.
The researchers then recorded another image without the virus present, and calculated the difference between the two images. This important process removes unwanted scattering by the surrounding environment.
The resulting images resolved details as small as 22 nanometers. This was enough to clearly define both the outer virus envelope and the capsid—the protein shell at the centre of the virus which contains the DNA.
Comparing contrast
The researchers recorded images of similar stained viruses with a scanning electron microscope (SEM) and an atomic force microscope (AFM). These techniques revealed the same general virus structure.
X-ray diffraction microscopy currently has much lower resolution than electron microscopes. However it has two significant advantages—it does not require staining of the viruses, and it provides higher contrast because the background scattering is removed.
Most promisingly, the x-ray images taken by Song and co-workers revealed electron density variations inside the viral capsid, which might provide details on the packing of the genome. The capsid has only been visualized before by laboriously taking averages over thousands of images from an electron microscope held at cryogenic temperatures.
This proves that x-ray diffraction microscopy can reveal subtle variations in structure that cannot be picked out by other techniques. It has other advantages, as Song explains: “Both electron microscopy and x-ray diffraction microscopy are powerful probes in a different sense. However, x-rays can probe thick specimens in air, whilst electron microscopes need a high vacuum environment and thin specimens.”
What’s more, x-ray diffraction microscopy does not need an imaging lens, eliminating the possibility of distortion or focusing limits in the equipment.
Free-electron future?
In theory, x-ray diffraction microscopy is limited only by the coherence of the available x-ray flux. Unfortunately when the x-rays get too strong they can start to do irreversible damage to biological samples.
To avoid damage the samples can be cryogenically cooled, but with standard x-rays there is still a practical limit of around five nanometers resolution. Even so, Song is not perturbed.
“Diffraction microscopy, or lensless imaging, can be applied with any coherent source, from photons to electrons to neutrons,” he says. “It can also be used with any wavelength.”
The most promising candidates for diffraction microscopy are new ‘fourth generation’ synchrotron light sources called x-ray free-electron lasers (XFELs). These devices use a relativistic beam of electrons to provide optical gain to a light signal.
Probing proteins
XFELs should produce coherent x-rays up to a billion times brighter than SPring-8’s. This could mean diffraction resolution down to the size of single atoms—leading to the ultimate goal of imaging proteins such as those in cell membranes.
“Presently, lots of membrane proteins are extremely hard to make as a single crystal,” explains Song. “This is a serious bottleneck given their importance in basic biological functions like signal transduction. X-ray diffraction microscopy may find its most competitive application in resolving the structures of those proteins.”
“RIKEN has the world’s best facilities and well-established research infrastructures which can make this cutting-edge research happen. Japan’s x-ray free-electron laser, one of the world’s three XFELs, is currently under construction at the RIKEN SPring-8 Center, and we plan to generate the first x-ray laser beam by March 2011. I am eager to use this facility for 3D imaging of single molecules at near-atomic resolution.”
References
- 1. Song, C., Jiang, H., Mancuso, A., Amirbekian, B., Peng, L., Sun, R., Shah, S.S., Zhou, Z.H., Ishikawa, T. & Miao, J. Quantitative imaging of single, unstained viruses with coherent x-rays. Physical Review Letters 101, 158101 (2008). doi: 10.1103/PhysRevLett.101.158101
About the Researcher
Changyong Song
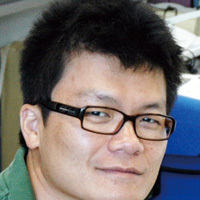
Changyong Song was born in Korea in 1972. After receiving a bachelor's degree from Physics Department of Jeonbuk National University in 1995, he went to the US in 1996 and obtained a Ph.D. in experimental condensed matter physics from Iowa State University (Ames, Iowa, USA) in 2001. On receiving the degree, he returned to Korea as a postdoctoral researcher, substituted service of his compulsory military duty and continued his research on resonant x-ray scattering at POSTECH until late 2004. Finishing the term in Korea, he moved back to the US as a postdoctoral researcher working on coherent x-ray diffractive imaging at UCLA. In March 2008, he joined RIKEN as an initiative research scientist at the Song Initiative Research Unit to develop an innovative x-ray microscope with the Japan X-ray Free Electron Laser (XFEL), one of the world's first three XFELs. Changyong Song aims to realizing near atomic resolution, single macromolecule 3D x-ray microscopy with XFELs.