Apr. 24, 2009 Research Highlight Biology
Unraveling fibers
High-resolution structural data about an essential protein reveal new insights into how some cells transform fiber into force
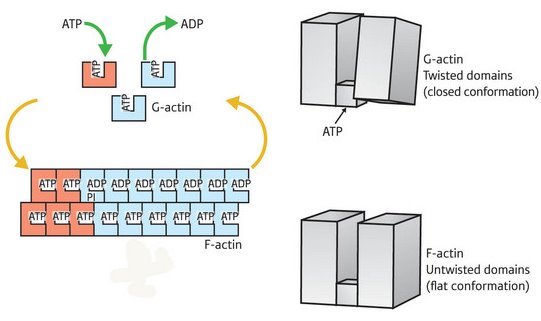
The actin protein exists in two major forms in the cell: as individual molecules of globular (G)-actin, or linked together as long filaments of fibrous (F)-actin. Actin microfilaments provide the primary scaffolding for contractile muscle fibers, and act as a key component of cellular infrastructure in general. However, many cell types also derive their mobility from directional microfilament growth and disassembly, a process powered in part by the hydrolysis of energy-providing adenosine triphosphate (ATP) molecules.
Although crude structural data on actin have been available for well over a decade, these have proven insufficient to provide a detailed understanding of the mechanism of the G-to-F-actin transition and ATP binding and hydrolysis. As such, a high-resolution structure of F-actin published recently in Nature by RIKEN SPring-8 Center researcher Toshiro Oda and his colleagues represents an important leap forward in understanding the function of this essential protein1.
Their findings revealed a major structural difference between the two states, an overall ‘flattening’ of the actin molecule as it enters the F state, brought about by a large shift in the relative positioning of the protein’s two segments. “A simple rotation of the two major domains of the actin molecule is the essence of the G- to F-actin transition,” explains Oda. “This simple rotation produces the flat conformation.”
In the current model for microfilament assembly, the association of ATP with F-actin has a stabilizing effect, while the hydrolysis of ATP by actin’s enzymatic subdomain is believed to destabilize the F-actin and induce localized disruption of the microfilament. The data from Oda’s team indicate that the flattening of F-actin not only drives fiber polymerization, but also leads to other internal rearrangements favorable to subsequent ATP hydrolysis (Fig. 1), although additional analysis will be required to confirm this.
“Our structure in this paper gives us the basis for understanding the biological processes in which actin participates,” says Oda, who adds that their data should prove a major asset for muscle researchers as a complement to the abundant high-resolution structural data on myosin—the other major protein component of muscle fibers.
Oda’s group, on the other hand, is following up with investigations into the ramifications of their structure for clarifying the process of actin-driven cell-motility. “We’re checking our ideas about ATP cleavage and energy usage,” says Oda. “Understanding the ATP usage is important for understanding the driving force of the ‘actin motor’.”
References
- 1. Oda, T., Iwasa, M., Aihara, T., Maeda, Y. & Narita, A. The nature of the globular- to fibrous-actin transition. Nature 457, 441–445 (2009). doi: 10.1038/nature07685