Sep. 18, 2009 Research Highlight Physics / Astronomy
Too many neutrons break the rules
Unusual behavior in an exotic isotope of neon challenges the traditional foundations of nuclear physics
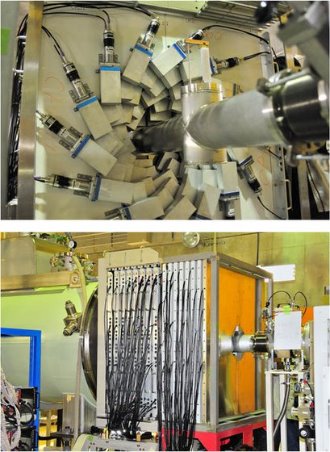
Nuclear physicists are fascinated by so-called ‘exotic’ nuclei, which are always on the edge of breaking apart because they contain too many, or too few, neutrons. Studying them, however, is problematic because exotic nuclei are very difficult to produce, and have a very short lifetime.
Now, physicists can work with a strong beam of exotic nuclei produced in the Radioactive Ion Beam Factory (RIBF) at the RIKEN Nishina Center for Accelerator-Based Science in Wako. The RIBF contains the world’s most powerful cyclotron, which can accelerate ions close to the speed of light and produce unstable isotopes that have never been studied before.
RIKEN’s Pieter Doornenbal and Heiko Scheit, and their co-workers from Japan, China, France and Germany have used the RIBF to produce and study an exotic isotope of neon (Ne), called 32Ne. They report that 32Ne belongs to a rare group of isotopes that are strongly deformed, and challenge the traditional foundations of nuclear physics1.
Magic numbers get tricky
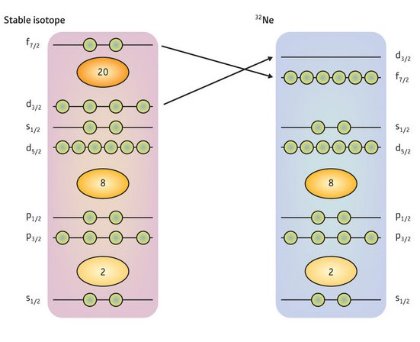
Protons and neutrons at the center of an atom are generally modeled as individual particles moving in particular orbits, or shells, similar to the electron shells orbiting around a nucleus. ‘Magic numbers’, which represent some particularly stable configurations of protons and neutrons, are an important concept in the nuclear shell model. The magic numbers are: 2, 8, 20, 28, 50, 82 and 126.
Atomic nuclei containing a magic number of protons or neutrons tend to be more tightly bound together, and are less likely to undergo nuclear decay, because there are large gaps in the energy spectra of orbits that these particles can occupy.
However, exotic nuclei disobey these rules, especially when they have many more neutrons than protons. For example, isotopes of neon, sodium and magnesium that contain 20 neutrons show no large energy gaps, even though 20 was traditionally a magic number.
“It was shown … that 20 is not a magic number anymore for these nuclei, which was a great surprise,” says Scheit. Nuclear physicists now refer to this group of misbehaving isotopes as the ‘island of inversion’.
“The island of inversion is a region in the nuclear chart where the standard ordering of single particle energies is inverted, meaning that the isotopes have a completely different structure from their counterparts nearer to stability.”
Targeting the island of inversion
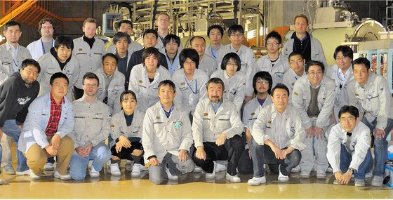
The island of inversion for neon begins at 30Ne, which has 20 neutrons, but no heavier neon nuclei have been studied until now. Scheit and co-workers were able to perform the first spectroscopic study of 32Ne (22 neutrons) thanks to the technology in place at the RIBF.
“The RIBF is a new-generation radioactive ion beam facility,” explains Scheit. “We wanted to study 32Ne for a long time, but we were not able to do so until the RIBF was completed [in 2007].”
The RIBF produces over a hundred times more 32Ne than any other facility in the world, mainly because its ion beams are far more intense and energetic.
In 1990 at the French facility GANIL, the rate of production of 32Ne was only four nuclei in two days, and in 1997 at RIKEN only 90 nuclei per day could be produced, according to Scheit. “Now, at the new facility, we have five nuclei per second!”
Finding neon
To produce 32Ne, the researchers direct a powerful beam of calcium-48 ions onto a rotating target made of beryllium. During this collision, if exactly ten protons and six neutrons are ‘knocked off’ a calcium ion then 32Ne is the remainder and continues down the beamline. “The probability of this happening is so low it takes 1011 (100,000,000,000) calcium-48 ions to produce one 32Ne nucleus!” says Scheit.
All the collision products are detected by the BigRIPS fragment separator—a cutting-edge machine and another key to the RIBF’s success. BigRIPS contains huge superconducting magnets that sort the different types of particle based on their charge and mass. Most are discarded, leaving a beam of separated 32Ne.
“The 32Ne in the beam is in its ground state,” explains Scheit. “To excite it we focus it onto a thick carbon target. After excitation, a gamma ray is emitted and the nucleus is again in the ground state. By measuring the energy of this gamma ray, we know the energy of the first excited state.”
After just eight hours of running their experiment (Fig. 1), the researchers detected enough gamma rays to prove that the energy of the first excited state of 32Ne is very low. There is no shell energy gap, meaning that 32Ne, like 30Ne, belongs in the island of inversion.
The results imply that32Ne nuclei are very deformed, despite being close to the magic number of 20 neutrons. The energy levels of individual protons and neutrons interfere with one another forming so-called ‘intruder configurations’.
“Normally the single particle energy levels are ordered in a certain way,” explains Scheit. “If that order is inverted, we speak of intruder configurations, because the normally upper levels now ‘intrude’ into the lower levels (Fig. 2).”
New candidates already in their sites
These findings come almost exactly 30 years after French researchers2 first noticed a much lower than expected energy of the first excited state in the magnesium isotope 32Mg. Scheit hopes that the world-leading facilities at the RIBF will help scientists unravel more mysteries about exotic nuclei.
“Soon we would like to extend our studies to 38Mg and also study other properties of 30Ne, 32Ne and 36Mg,” he says. “All these studies are currently only possible here at the RIBF.”
References
- 1. Doornenbal, P., Scheit, H., Aoi, N., Takeuchi, S., Li, K., Takeshita, E.,Wang, H., Baba, H., Deguchi, S., Fukuda, N. et al. Spectroscopy of 32Ne and the “island of inversion”. Physical Review Letters 103, 032501 (2009). doi: 10.1103/PhysRevLett.103.032501
- 2. Détraz, C., Guillemaud, D., Huber, G., Klapisch, R., Langevin, M., Naulin, F., Thibault, C., Carraz, L.C. & Touchard, F. Beta decay of 27-32Na and their descendants. Physical Review C 19, 164–176 (1979). doi: 10.1103/PhysRevC.19.164
About the Researcher
Heiko Scheit
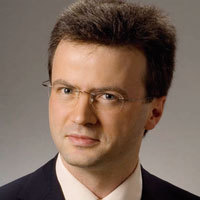
Heiko Scheit was born in Germany in 1971. After completing basic physics training at the Friedrich-Schiller-University in Jena, Germany, he went to Michigan-State-University (MSU) in Michigan, USA, where he specialized in nuclear physics and obtained his MS (1997) and PhD degrees (1998) carrying out research projects at the National Superconducting Cyclotron Laboratory (NSCL) located on the MSU campus. Next he went to Max-Planck-Institute for Nuclear Physics in Heidelberg, Germany, where, after two years of postdoctoral experience, he became the nuclear physics group leader in the division of Dirk Schwalm. After a short visit to the NSCL, he took up a permanent staff position at the RIKEN Nishina Center in the Radioactive Isotope Physics Laboratory. His research focuses on the experimental study of the structure of light exotic nuclei far from stability, which are produced at the newly commissioned Radioactive Ion Beam Factory of the RIKEN Nishina Center.