Jun. 3, 2011 Research Highlight Chemistry
A diamond ring sparks a paradigm shift
Trapping four silicon atoms into a short-lived, diamond-shaped complex gives surprising insights into aromaticity
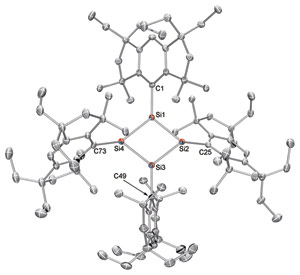
The sweet smell of benzene gave birth to the term ‘aromatic’ molecules, but it is the chemical bonds within these compounds that have fascinated researchers for almost 200 years. Encasing alternating double- and single-bonded carbon atoms inside a flat ring allows so-called ‘pi’-electrons to delocalize and move around the cyclic framework. And thanks to the curious rules of quantum mechanics, this pi-electron sharing has radical consequences for differently sized rings. While aromaticity makes hexagonal systems like benzene exceptionally stable, ‘anti’-aromaticity makes four-membered rings like cyclobutadiene show opposite tendencies—the delocalized electrons try to rip the molecule apart.
To this day, understandings of anti-aromatic molecules remain controversial. Katsunori Suzuki, Tsukasa Matsuo, Kohei Tamao and colleagues from the RIKEN Advanced Science Institute in Wako created their own controversy when they attempted to gain a new perspective on these compounds by synthesizing the first analog of cyclobutadiene made from silicon (Si) atoms. After racing against time to characterize the unstable specimen, the team stared at their analytical results in disbelief. It appeared the four silicon atoms had arranged into a flat planar rhomboid (Fig. 1)—a diamond-shaped ring —that had been predicted theoretically, but never observed in reality.
“When we found the diamond-like Si4 structure, we were very surprised,” says Suzuki. “What is this? Why is it rhombic?” The team’s answers to these questions, published recently in Science1, shed light on how atoms rearrange to avoid pi-electron repulsions, and set the stage for new explorations of pi-conjugated silicon materials.
Putting the pieces together
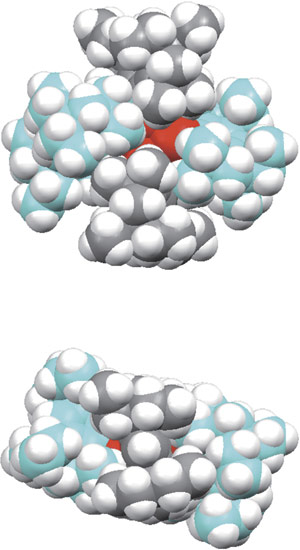
Isolating cyclobutadiene-based compounds is a tricky affair that typically requires extremely cold temperatures, not far from absolute zero, to trap the short-lived substances. Producing a silicon version of cyclobutadiene is especially daunting. The pi-electrons in silicon double bonds are held less tightly than in carbon units, making this species even more reactive.
Fortunately, the researchers recently developed molecules known as substituted s-hydrindacenes, or ‘Rind’ groups for short, that stabilize silicon double bonds in ways never seen before. With an inner skeleton of three fused hydrocarbon rings surrounded by finger-like alkyl chains, Rind groups can fit together like puzzle pieces and lock silicon double bonds into planar crystals with unique light-emitting capabilities2.
“In sharp contrast to previously developed protecting groups, our Rind groups are size-controllable, easy to prepare, and have tunable solvent compatibility,” says Matsuo. “In light of our previous observations, we anticipated that Rind groups could stabilize a planar cyclic pi-conjugated silicon system.”
By using a lithium reagent to add electrons to a Rind-substituted silicon precursor called (EMind)SiBr3, and employing meticulous care to exclude any air or moisture from the reaction, Suzuki and colleagues successfully isolated a small amount of pure orange Si4(EMind)4 crystals. X-ray analysis revealed an unprecedented Si4 planar rhomboid, imprisoned by four Rind groups meshed together like a molecular gear (Fig. 2).
Observing the shift
Ideally, cyclobutadiene should appear as a flat square, with carbon atoms on each corner. However, theoretical and experimental studies have shown that cyclobutadiene breaks its molecular symmetry, through a process known as a covalent Jahn-Teller distortion, and reshapes itself into a rectangle to minimize anti-aromatic repulsions. To determine why the Si4 analog crystallized into a diamond arrangement, the researchers turned to nuclear magnetic resonance (NMR) spectroscopy, a highly sensitive technique for monitoring molecular conformational changes.
Running NMR experiments, however, proved difficult because Si4(EMind)4 is thermally unstable, with a half life of only three days at 25°C. Nevertheless, the team persevered and observed that, in a room temperature solution, the diamond-like Si4 skeleton was actually shifting between two longitudinal and lateral rhombic isomers at an extremely swift speed. This geometric conversion, which was facilitated by the gear-like Rind groups, persisted to low temperatures until crystallization occurred.
Squaring off against aromaticity
Next, the team employed sophisticated theoretical calculations to gain an electronic understanding of their new structure. These computations indicated that several isomers, including rhombic, rectangular, and square structures, could result from a Si4(Rind)4 complex. In agreement with the experiments, however, the researchers saw that planar rhomboid was the most stable isomer energetically.
While silicon double bonds usually share their pi-electrons equally, the researchers found that the rhombic shape arises from a Jahn-Teller distortion that polarizes electronic charges. By separating the unstable pi-electrons into alternating positive and negative charges on each corner of the silicon diamond, the molecule can convert from an anti-aromatic to a non-aromatic state. This remarkable charge-separation was confirmed by the appearance of two peaks in solid-state silicon NMR measurement—clear evidence that identical silicon atoms experienced two separate electronic environments.
“Synthesizing a rhombic-shaped cyclobutadiene is quite difficult, because the carbon–carbon pi-bond is very strong” explains Matsuo. But since silicon double bonds are weaker and therefore more flexible, they can stretch apart to generate the diamond-like arrangement. “The exquisite geometric effects of Rind groups and the elemental character of silicon atoms make the rhomboid analog possible,” he says.
These findings not only reveal some fundamental differences between silicon and carbon atoms, but also how they can be overcome. For instance, by modifying the bulkiness of the Rind ligands, the team is confident that a hexagonal benzene-like silicon compound could soon materialize.
According to Matsuo, this diamond-shaped ring also provides a starting point for new functional materials based on pi-conjugated silicon. “One of our target materials is a polyacetylene–silicon analog,”—a compound with potent light-emitting and electron-conducting capabilities—“and we now have a chance to make it with the power of Rind ligands,” he says.
References
- 1. Suzuki, K., Matsuo, T., Hashizume, D., Fueno, H., Tanaka, K. & Tamao, K. A planar rhombic charge-separated tetrasilacyclobutadiene. Science 331, 1306–1309 (2011). doi: 10.1126/science.1199906
- 2. Kobayashi, M., Matsuo, T., Fukunaga, T., Hashizume, D., Fueno, H., Tanaka, K. & Tamao, K. Air-stable, room-temperature emissive disilenes with π-extended aromatic groups. Journal of the American Chemical Society 132, 15162–15163 (2010). doi: 10.1021/ja108094m
About the Researcher
Tsukasa Matsuo (left), Kohei Tamao (center) and Katsunori Suzuki (right)
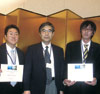
Kohei Tamao received his Dr of Eng. from Kyoto University under the direction of Prof. Makoto Kumada in 1971 after which he rose steadily through the ranks of the same institution becoming Full Professor in 1993. In 2005, he moved to RIKEN as Director of the Frontier Research System and is currently Director of the RIKEN Advanced Science Institute and a unit leader of the Functional Elemento-Organic Chemistry Unit at RIKEN. He received the American Chemical Society Frederic Stanley Kipping Award in 2002. His current research interests are organosilicon and related elemento-organic chemistry.
Tsukasa Matsuo graduated from Tohoku University with BSc (1994) and MSc (1996) degrees under the direction of Prof. Hideki Sakurai and Prof. Mitsuo Kira. He received his PhD (1999), supervised by Prof. Akira Sekiguchi, from the University of Tsukuba and became Assistant Professor at the Tsukuba Advanced Research Alliance in 1999. In 2001 he moved to the Institute for Molecular Science as Assistant Professor and in 2007 was appointed Deputy Unit Leader at RIKEN. His research interests are the structures, reactivities and physical properties of elementoorganic compounds.
Katsunori Suzuki graduated from the Faculty of Science, Tohoku University, with a BSc in 2002. He subsequently took his MSc (2004) and PhD (2007) degrees under the direction of Prof. Mitsuo Kira from the same university. In 2007, he moved to the Functional Elemento-Organic Chemistry Unit at RIKEN as a Research Scientist. His current research interests include synthetic approaches to cyclic π-conjugated aromatic/antiaromatic systems containing heavy group 14 elements, and the development of synthetic methodologies for π-conjugated silicon-silicon unsaturated frameworks for functional elementoorganic materials.