Jul. 5, 2014
Development of high-efficiency deep-ultraviolet LED
RIKEN No.: 07745, 07323, 08095
Inventors
Hideki Hirayama (Quantum Optodevice Laboratory)Background
Since the light in wavelength region around 260 nm (deep-ultraviolet region) in the ultraviolet region is expected to be useful in a wide range of applications including disinfection, water purification and medicine, development of deep-ultraviolet LEDs (DUVLEDs) is popularly being conducted. The typical construction of deep-ultraviolet LEDs includes a sapphire substrate equipped with aluminum gallium nitride-type semiconductor multilayer structure mainly comprising of Al, Ga and N.
The external quantum efficiency of an LED is expressed as the multiplication value among internal quantum efficiency (IQE), electron injection efficiency (EIE) and light extraction efficiency (LEE).
[Formula] ηext (external quantum efficiency) = ηint (internal quantum efficiency) × ηinj (electron injection efficiency) × ηlee (light extraction efficiency)
It is extremely difficult to develop a deep-ultraviolet/ultraviolet LED in the 250-330 nm band which has a low hole concentration and insufficient electron barrier height, and studies are being conducted to address high efficiency and high output.
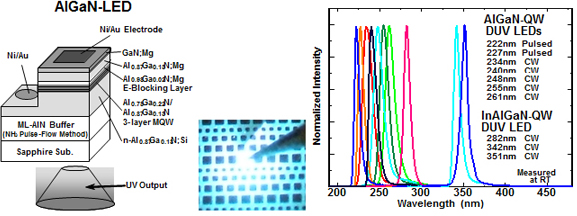
Fig.1:Realization of short wavelength semiconductor light source (λ=222-351nm)
Summary
[Development of AIN buffer with the world’s highest quality]
Conventionally, the low luminous efficiency of the AIN buffer had been the greatest cause of the low external quantum efficiency of LEDs. While the band-gap energy is enhanced by increasing the Al composition of AlGaN in preparation of the solid light emitting device on the short-wavelength side, it also causes the internal quantum efficiency to drop, as the density of threading dislocation in the thin semiconductor layer increases due to the inconsistency between the sapphire substrate and lattice constant.
Polarity control becomes extremely important in group III nitrides such as AlGaN. Therefore, we have found that stable group III polarity can be addressed by pulse-supplying NH3, and completed “ammonia pulse-flow multilayer growth method.” AIN buffer prepared by this method reduced the threading dislocation rate to 1/100 of the conventional level, and it also addressed crack generation prevention and atomic layer flattening. The internal quantum efficiency in the ultraviolet region (280 nm) was improved dramatically from the conventional below 0.5% to 50% and emission intensity to 80-fold.
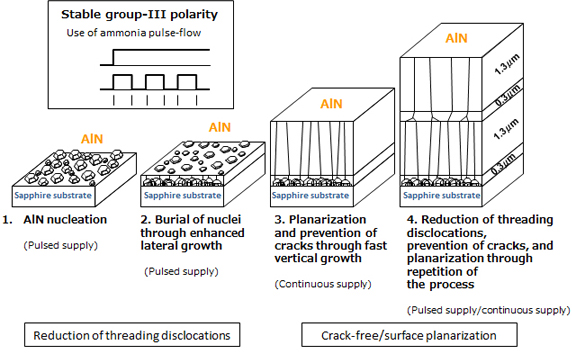
Fig.2: Progress of crystal growth technology by NH3 pulse-flow multilayer growth method
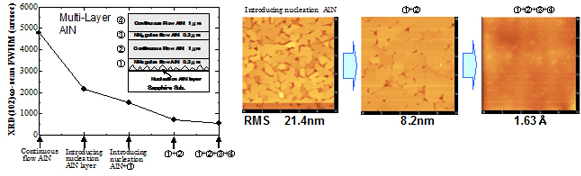
Fig.3: Realization of low threading dislocation density and atomically flat surface in AlN buffer layer
[Dramatic improvement in emission intensity by multiquantum barrier (MQB)]
Conventionally, improvement in electron injection efficiency was extremely difficult, as the hole concentration of P-type AlGaN was low. While the InAlGaN-type ultraviolet device uses AlGaN with a similar composition to AIN, it is difficult in principle to form a barrier with equivalent or higher than that.
Since the practical electron block height increases to 2 - 3 times by using multiquantum barrier (MQB) due to the multiple reflection effect of the electron, the electron block effect is increased compared to the case of the single barrier. In this invention, the electron injection efficiency was improved dramatically from 20% to 80% by using MQB.
The electron blocking effect by MQB becomes better as the barrier height is larger in either the case of growth on plane A or the case of crystal growth with polarity on plane C. Furthermore, by changing the MQB cycle in order, it becomes possible to block electrons over a wide energy range and electron blocking effect becomes even more prominent than the MQB of the same cycle. Because of this invention, it has now become possible to provide the elemental technology for further increasing the emission intensity of deep-ultraviolet light using AlGaInN-type materials, especially AlGaN-type materials, as the luminous layer materials.
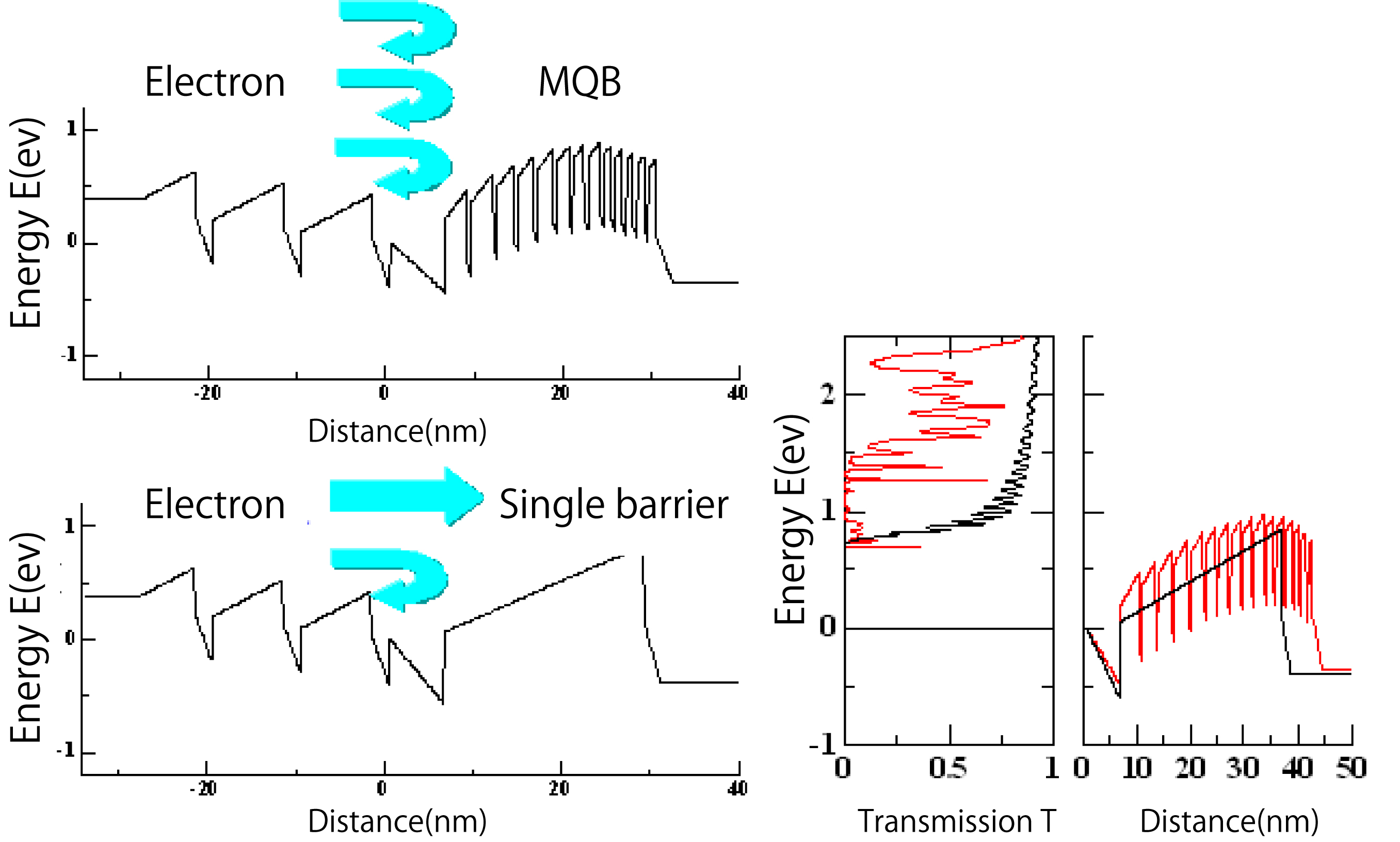
Fig.4: Concept of the effect by multi quantum barrier (MQB) and example of the analysis
[Development of high-output LED with connected-pillar AIN buffer]
To improve the external quantum efficiency while suppressing the effects on internal quantum efficiency or light extraction efficiency as much as possible, a buffer layer with low dislocation that can deliver light direction conversion effect is necessary. We therefore prepared an AIN buffer layer with a structure called connected-pillar AIN buffer.
The connected-pillar AIN buffer has a structure comprising of the pillar alignment block in which multiple pillars are aligned and the unified section which is formed as the pillars are joined. Each pillar extends from each projecting part of the sapphire substrate in the normal direction on one plane and is partitioned from others by gaps in the in-plane direction. The projecting part of the sapphire substrate has the effect to facilitate crystal growth into each pillar from the apex.
The buffer layer of this connected-pillar AIN crystal delivers the light direction conversion effect as a crystal structure with low dislocation is formed by the crystal structure of the unified section with connected-pillars and it has the structure with pillars and gaps. This invention addressed deep-ultraviolet/ultraviolet LED with improved light extraction efficiency.
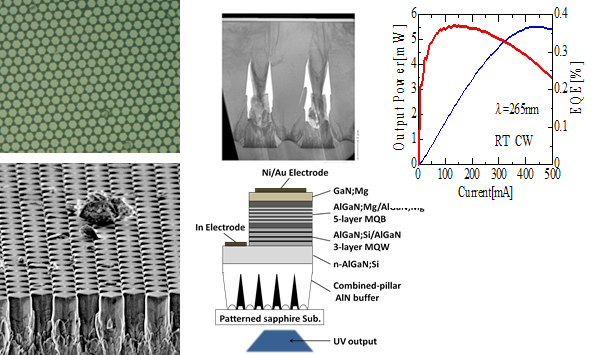
Fig.5: The world's first success of creating connected-pillar AIN buffer
Merits
- Wide ultraviolet wavelength range (wavelength: 200-360 nm)
- High-efficiency light emission possible using quantum well
- p-type and n-type transmission possible
- Hard materials (addresses long-life devices)
- Arsenic-, lead-and mercury-free materials (harmless to the environment)
Applications
[Disinfection] Wavelength: 270nm
Direct disinfection without heating (no development of resistant bacteria, no deterioration)
- Disinfection in household applications (such as refrigerators, water purifiers, air conditioners, air purifiers, humidifiers, thermos bottles, circulating baths, tinea pedis treatment devices, and vacuums)
- Disinfection of distribution channels for food/agricultural products and livestock products (such as eggs, boiled fish pastes, belt conveyors, pet bottle mouths, and packing materials for drugs)
- Disinfection equipment for large facilities (such as waterworks tanks and air equipment)
[Medical application] Wavelength: 250 - 280 nm, 300 - 320 nm, etc.
- Skin affliction treatment
- Laser scalpels, cell sorting
[High-speed decomposition process for pollutants] Wavelength: 260 - 320 nm
- Purification of pollutants with titanium oxide (photocatalyst reaction) (such as dioxins, PCBs and environmental hormones)
- High-speed purification of automobile exhaust gas (pollution-free cars)
[High-density optical recording lasers] Wavelength: - 250 nm
- DVD for deep-ultraviolet
[High color rendering and long-life fluorescent lamps] Wavelength: 340 nm
- White light UV-LED array with high luminance
- High efficiency (~40%), long life (several decades)
[Ultraviolet curing resin, biochemistry industry] Wavelength: 250 - 280 nm, 365 nm, etc.
[Various light information sensing] Wavelength: 250 - 280 nm, 325 nm, etc.
- Fluorometry, surface analysis, ultraviolet sensor, etc.
Future goals
- Development of a deep-ultraviolet LED with efficiency of about 20% is planned in about 2 years.
- Possibilities of developing the watt-class high-output device with device integration
- Popularization for disinfection and water purification purposes in homes, hospital facilities and food distribution and so forth
References
- 1.Patent No.5120861, U.S. Patent No.7888154
- 2.U.S. Patent No.8759813
- 3.U.S. Patent No.9660140