Jun. 21, 2019 Feature Highlight Chemistry Computing / Math
Now polymers can self-heal, even when wet
New spontaneously self-healing polymers don’t mind a bit of wetness, and they may be useful in everything from dentistry to liquid crystal display (LCD) screens
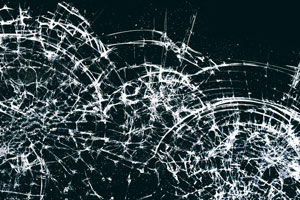
Imagine if a crack in your smartphone screen disappeared in minutes. At RIKEN, several new polymers that can heal with great strength, without outside energy input, and even in water, or acidic and alkaline solutions, hint at this possibility.
It's the latter three conditions that sets us on the path of everyday use in self-healing liquid crystal display (LCD) screens. While self-healing materials have been on the radar for more than a decade, most conventional self-healing polymers face at least two barriers: one, some kind of energy, such as heat or pressure, needs to be applied to stimulate healing, and, two, these materials don’t heal well when wet.
Both of these problems are linked to fact that these materials typically manipulate particular bonds, such as hydrogen bonds, covalent bonds or ionic interactions—covalent bonds require extra energy to re-form, and hydrogen and ionic bonds can be broken in water, and acidic or alkaline liquids.
Now, a team led by Zhaomin Hou of the RIKEN Center for Sustainable Resource Science, has developed a number of self-healing polymers in which physical bonds spontaneously reform when ruptured. And, because these bonds are unique, contact between the polymer and water, acid or base solutions—as is the case in liquid crystal display screen constuction—doesn’t inhibit self-healing.
The scientists have been able to create a number of polymer materials, including soft viscoelastic materials, tough elastomers—materials able to be stretched and return to their original shape—and rigid plastics.
Initially, the stretchy elastomers have shown the most promising self-healing properties, says Hou. For example, in one test, when one of the elastomer polymers was cut in two with scissors, when held together the parts recombined within minutes. After five days of self-healing at room temperature, this process had created bonds so strong that the polymer didn’t break along the cut when stretched to several times its original length. The authors describe this measured strength as “the highest ever reported for an autonomously repaired material…even higher than most pristine autonomous self-healing materials reported to date.”
Using physical force
The secret lies in the polymers’ structure, composed of long, spaghetti-like molecules that are formed by two monomers—the building blocks from which polymer molecules are made. One is ethylene (C2H4), the same monomer that makes up polyethylene, the most popular plastic in the world, used to make plastic bags, shampoo bottles and toys. The other monomer is known as anisylpropylene.
Normally, it would be next to impossible to combine these two monomers in the desired manner. They are not usually attracted to each other, as anisylpropylene is polar, meaning that it contains units in which one end is slightly positively charged and the other end is slightly negatively charged, while ethylene is non-polar. Usually, anisylpropylene is also much less reactive than ethylene for polymerization.
Hou’s team was able to overcome the reluctance of the two monomers to combine by using a catalyst—tiny amounts of a rare-earth metal called scandium. They also combined the two monomers in a controlled manner, so that by varying the initial conditions, they could make a whole series of polymers with slightly different properties.
The copolymers created consist of relatively long segments, in which ethylene alternates with anisylpropylene, and these are punctuated by shorter strings of ethylene units. Weak physical bonds form between the alternating segments, as well as between the ethylene segments. Consequently, when these bonds are ruptured by cutting the polymer, they will try to reform, and it is this that creates the elasticity and self-healing properties of the polymer.
Plenty of real-world possibilities
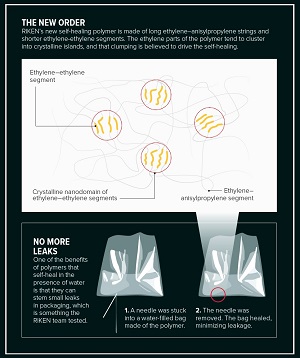
Hou and his team didn’t set out to make self-healing polymers. “It was a serendipitous discovery,” he says. “We knew we could make new structures, but we had no way of predicting what their mechanical properties would be. It was only after we made the polymer and checked its properties that we discovered it could self-heal.”
Even after making the polymer, Hou’s team were in the dark about how it was healing itself. “We’re synthetic chemists, and so it was challenging for us to understand the physical properties of the resulting polymer,” explains Hou. “To pin down how it was self-healing, we had to collaborate with some polymer physics specialists at Kyushu University.”
The teams were soon demonstrating just how useful the new polymers could be. For example, the team made a bag from one polymer and filled it with water. When punctured with a needle, this polymer’s self-healing process was so fast that little water dripped out as the needle was removed and the bag self-healed. This, says Hou, could pave the way for more robust or reusable food packaging or blood transfusion bags.
Their elastomer polymers are also strong and have good elasticity, one able to be stretched to 20 times its original length. Both the flexible and rigid plastics the teams created had excellent shape memory, meaning that they can return to their original shapes after being stretched and distorted at a high temperature and cooled and then heated. It will mean these polymers can be molded into industrially useful forms, explains Hou.
“The potential is huge,” he enthuses. “There are many, many potential applications.” His team has already received enquiries from companies in various fields ranging from automobiles to cell phones, and medical care. But Hou says the first step will be to team up with a chemical company to work out how to produce large quantities of the polymers, which he says should be able to be made relatively cheaply. The group will also continue to try using other combinations of monomers to see what other kinds of polymers they can materialize.
Video
References
- 1. Wang, H., Yang, Y., Nishiura, M., Higaki, Y., Takahara, A. & Hou, Z. Synthesis of self-healing polymers by scandium-catalyzed copolymerization of ethylene and anisylpropylenes. Journal of the American Chemical Society 141, 3249–3257 (2019). doi: 10.1021/jacs.8b13316
About the Researcher
Zhaomin Hou
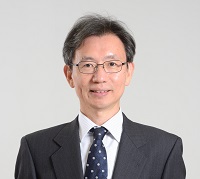
Zhaomin Hou obtained his PhD in chemistry from Kyushu University in Japan in 1989. After postdoctoral training at RIKEN and the University of Windsor in Canada, he joined RIKEN in 1993. Since 2013, he has worked as the Deputy Director of the RIKEN Center for Sustainable Resource Science (CSRS), concurrent to his work as Group Director of the Advanced Catalysis Research Group at the CSRS and the Director of the Organometallic Chemistry Laboratory at RIKEN. His research interests include the activation and utilization of small molecules such as nitrogen gas and carbon dioxide and the development of more efficient, selective catalysts for synthesizing functional molecules and polymers.