Dec. 25, 2020 Feature Highlight Physics / Astronomy Computing / Math
A one-way street for sound waves
A new way for sound waves to interact with magnetic film could lead to 80% more directional control.
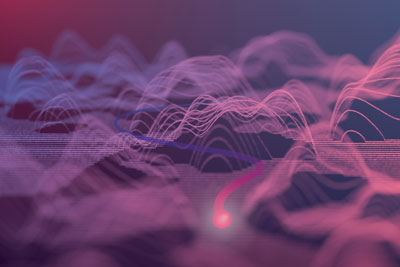
We are one step closer to devices that can control the direction and power of sound waves. A team led by Yoshichika Otani at the RIKEN Center for Emergent Matter Science (CEMS) has fabricated a magnetic film in which sound waves interact with electron spins, so that the amplitude and direction of sound waves can be manipulated with a similar degree of control as electrical currents.
The interaction involves ‘magneto-rotation coupling’, which is when the rotational motion of surface sound waves causes the atomic lattice in a ferromagnetic material to move in a circular motion. The magnetism of a ferromagnet, the classic example of which is the bar magnet, derives from the spins of their electrons tending to align in the same direction. Coupling makes the magnetization precess, a wobbling motion that occurs when a spinning object is the subject of an external force. Depending on the direction of the sound waves, this precession can weaken the waves or leave them unaffected. An exciting finding is that when conditions are tweaked in accordance with theory, the sound waves travel in only one direction.
One direction tune
It was only during the review process of their research paper that the team realized that they had achieved 100% ‘rectification’. Rectifiers are essentially one-way streets that allow energy to flow in a single direction. One of the reviewers noted theory predicted that complete rectification should be possible, and when the team re-examined their data, they found that their measurements did indeed show 100% rectification — a big increase over the previous record of 20%.
Importantly, the amount of rectification can be easily controlled. “The magnetic field controls how much you block the sound waves traveling in one direction,” says Jorge Puebla, one of the co-authors from CEMS. “So you could have a knob on your device that adjusts how much sound comes in from the outside and how much you block, for example.” Puebla notes that the sound waves used in this experiment are too high in frequency to be audible to people, so this will require further study for more conventional sound blocking uses. However, this finding could be more immediately useful in sound isolators for other types of experiments.
In addition, electrical rectifiers that permit electric currents to flow one direction but not the other are used extensively in the electronics industry. For example, they can be used to convert alternating electric currents into steady direct currents. Now, acoustic rectifiers based on magneto-rotation coupling are a possibility, and might be useful in devices such as sound isolators and specialized magnetic properties sensors, which could be applied to superconductivity research among other areas.
One direction tune
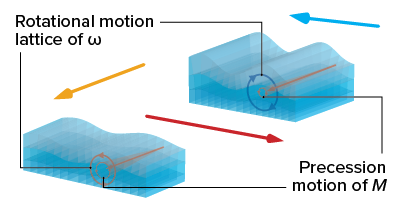
It was only during the review process of their research paper that the team realized that they had achieved 100% ‘rectification’. Rectifiers are essentially one-way streets that allow energy to flow in a single direction. One of the reviewers noted theory predicted that complete rectification should be possible, and when the team re-examined their data, they found that their measurements did indeed show 100% rectification — a big increase over the previous record of 20%.
Importantly, the amount of rectification can be easily controlled. “The magnetic field controls how much you block the sound waves traveling in one direction,” says Jorge Puebla, one of the co-authors from CEMS. “So you could have a knob on your device that adjusts how much sound comes in from the outside and how much you block, for example.” Puebla notes that the sound waves used in this experiment are too high in frequency to be audible to people, so this will require further study for more conventional sound blocking uses. However, this finding could be more immediately useful in sound isolators for other types of experiments.
In addition, electrical rectifiers that permit electric currents to flow one direction but not the other are used extensively in the electronics industry. For example, they can be used to convert alternating electric currents into steady direct currents. Now, acoustic rectifiers based on magneto-rotation coupling are a possibility, and might be useful in devices such as sound isolators and specialized magnetic properties sensors, which could be applied to superconductivity research among other areas.
A familiar name
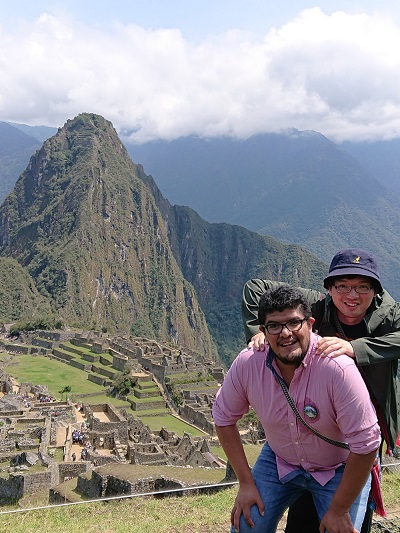
Ferromagnetism is an old technology, dating back at least two and a half millennia. But what has made this new control possible is the advancement of the emerging field of spintronics. The technology of spintronics uses the spins of electrons rather than their charges (as in conventional electronics) to convey information. It’s seen as promising for creating devices that have ultralow power consumption, as spin can flow without electrons physically moving. One way to create and manipulate the flow of spins, or spin currents, is to use sound waves in ferromagnetic materials. Sound waves that travel as ripples along the surface of a ferromagnetic material displace the electron spins, giving rise to a spin current. Known as magnetoelastic coupling, this effect has been extensively studied for some time.
However, the team was surprised when they scoured the literature for the magneto-rotation coupling they had observed. They uncovered a theoretical paper in the proceedings of a 1976 conference that predicted the effect, and the name of the first author of the paper, Sadamichi Maekawa, looked very familiar. In fact, he was a CEMS colleague who was working two floors below the team’s lab. The experimental observation of an effect that he predicted more than 40 years ago was sweet vindication for Maekawa: “I had almost forgotten about my work, but I was very happy when Dr Puebla discovered my paper.” Puebla concurs: “It was a very nice surprise to discover that we had someone to talk to who was so nearby.”
One reason it had taken so long to observe the effect is that the experiment needed to be performed at high frequencies, which requires small structures. Recent advances in nanofabrication have made it possible to make devices small enough to perform the measurement. “If you want to analyze this effect you need a really high-frequency device,” explains Mingran Xu, who did most of the experiments. “But you need extremely small structures to fabricate a high-frequency device. It was really quite challenging to make.”
The team now intends to use magneto-rotation coupling to explore magnetism in exotic two-dimensional materials. “Two-dimensional materials with magnetism have been discovered and some of them have magnetism out of the plane,” says Puebla. “But when you consider that these materials are just one or two atoms thick, it’s not really clear where this anisotropy is coming from. We want to fabricate some devices and see if we can contribute to this important question in two-dimensional materials."
Reference
- 1. Xu, M., Yamamoto, K., Puebla, J., Baumgaertl, K., Rana, B., Miura, K., Takahashi, H., Grundler, D., Maekawa, S. & Otani, Y. Nonreciprocal surface acoustic wave propagation via magneto-rotation coupling. Science Advances 6, eabb1724 (2020). doi: 10.1126/sciadv.abb1724
About the researchers
Mingran Xu, Student Trainee, Quantum Nano-Scale Magnetism Research Team, RIKEN Center for Emergent Matter Science
Mingran Xu is a PhD course student at Graduate School of Frontier Sciences at University of Tokyo and a research student at RIKEN. He was first supported by the RIKEN International Program Associate (IPA) Program, and later by a three-year Japan Society for the Promotion of Science fellowship (JSPS DC1). He has been working on a spin conversion project since began his masters. His research is focused on magnon–phonon coupling systems.
Jorge Puebla, Research scientist, Quantum Nano-Scale Magnetism Team, RIKEN Center for Emergent Matter Science
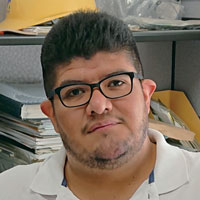
Jorge Puebla studied spin phenomena in semiconductor nanostructures (quantum dots and wires) in the Department of Physics and Astronomy at the University of Sheffield in the United Kingdom. Later, he was the recipient of a Marie Curie Fellowship and worked as the project leader in technology developments in microscopy, interferometry and photovoltaics for the German company Attocube Systems. In 2015, he joined RIKEN, where he leads projects on photon coupling and phonons with spin systems.