Jun. 28, 2022 Perspectives Biology Chemistry Engineering
Nature’s own assembly line
RIKEN-engineered microbes and plant lignin could soon help produce everything from car tires to engine components.
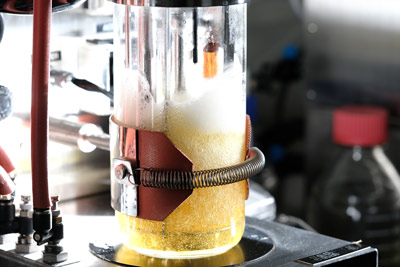
Today the raw ingredients for virtually all industrial products, ranging from medicines to car tires, come from non-renewable chemical feedstocks. They are produced in fossil fuel refineries that emit greenhouse gases, such as carbon dioxide. However, future chemical factories might invert this dynamic, manufacturing some compounds using plants that naturally construct complex chemicals by drawing carbon dioxide molecules from the air.
Tomokazu Shirai taps into biology’s native chemical capabilities, redirecting them so that plants and microbes cleanly produce the kinds of industrial chemicals currently derived from cracking crude oil. The synthetic biologist is senior scientist at the Cell Factory Research Team and joined the RIKEN Center for Sustainable Resource Science (CSRS, formerly, the RIKEN Biomass Engineering Program) in 2012. His team has already created the world’s first microbes that take glucose and convert it into maleic acid or 1,3-butadiene. These valuable industrial chemicals are used in myriad products, including polymers and rubbers1,2.
But this is just the first step for CSRS synthetic biologists. These engineered microbes need to be fed sugars to produce the target chemicals, but if plants are used as the host organism, their ability to assimilate carbon dioxide directly from the atmosphere will result in the carbon-negative production of many valuable chemicals.
Computer-aged design
Synthetic biology is an emerging area of research that combines chemistry, biology and engineering to rework the molecule-producing metabolic pathways of target organisms so that they produce valuable chemicals. CSRS scientists have expertise in catalytic chemistry and in chemical biology, but also many that specialize in large-scale data science, calculation and simulation, and AI.
The use of AI represents a departure from the traditional ways of doing synthetic biology. But this computational approach has been key to a collaboration with tire manufacturer, Yokohama Rubber, and Zeon Corporation. The joint venture has designed and created E. coli microbes that take glucose and convert it into 1,3-butadiene, a key synthetic chemical used to manufacture tires.
The first step in any synthetic biology project is to analyse the potential host’s metabolic pathways to identify points that could be diverted to produce the desired chemical. Any modifications must not kill or significantly impair the growth of the host.
Since 2012, Shirai has been developing and refining the simulation tool BioProV to navigate this complex biochemical space. BioProV is an AI trained in metabolic pathway classification and enzyme reaction patterns that analyses the natural metabolic pathways of an organism. It proposes pathway modifications to produce a target chemical without affecting the host’s overall metabolism. This in silico tool enables the design of artificial metabolic pathways and evaluation of their feasibility.
His team identified that E. coli naturally produces a molecule called muconic acid, which could be turned into 1,3-butadiene in two enzymatic reactions. To give the microbe the capacity to carry out the two missing steps, Shirai and his colleagues engineered enzymes for the necessary chemical conversion in 2021.
To do this, they identified known enzymes that could catalyse related reactions, and then modified them for the new reactions. Computational simulation was necessary to redesign and remodel the candidate enzymes’ active sites to accept the new substrate. The team rationally designed enzymes that achieved a 1,000-fold increase in activity compared to the original wild-type enzyme.
The DNA codes for these improved enzymes were inserted into the E. coli genome and now the 1,3-butadiene produced by these engineered microbes is readily piped from their bioreactor. The project’s commercial partners are currently scaling up the process to produce the kilogram quantities of 1,3-butadiene needed to manufacture and evaluate tires made using the bio-derived chemical.
Chemical companies employ many chemists, but few biological researchers, so to connect and collaborate with these companies to translate synthetic biology into the real world is a huge step.
Woodwork
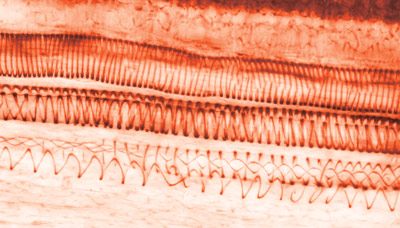
A sustainable alternative to traditional fossil fuel-derived chemical production is to take materials currently considered as waste, and chemically or biologically convert them into valuable products.
The woody stems and stalks of plants left over after the harvest of fruits and grains is one global-scale waste stream. The main component of these inedible plant parts is lignin, a tough biopolymer. Lignin is the most abundant compound from plants and one of the most abundant compounds on Earth. It can be sourced from agricultural waste and is the most inexpensive and sustainable source of carbon with which to make renewable fuels and chemicals. To use it as a feedstock for high-value chemicals could be highly beneficial to society.
Lignin’s complex chemical structure makes it difficult to break down and reassemble into new compounds. For example, a heat treatment known as fast pyrolysis can break lignin down into subunits called cinnamic monomers. These molecules feature a double bond that could potentially be used to recombine the monomers into advanced functional polymers. However, side chains located around the double bond impedes chemical reactivity, hindering efforts to make polymers from this biowaste.
CSRS scientist Hideki Abe recently developed a method to overcome this limitation3. Rather than synthetic biology, Abe used organocatalysis to clip cinnamic monomers together. Organocatalysis is a sustainable chemistry technique, recognized by the 2021 Nobel Prize for Chemistry, which uses small organic molecules as catalysts in place of traditional catalysts based on rare or toxic metals.
The resulting acrylic resins showed high strength, and resistance to heat and chemical degradation, suggesting a wide array of potential uses, including for car body and engine components.
Seeding future growth
Another waste product in abundant supply is atmospheric carbon dioxide.
For the Cell Factory Research Team, the next major challenge is using synthetic biology to develop plants that could absorb that carbon dioxide from the atmosphere and turn it into industrially important chemicals.
Compared to single-celled microbes, multicellular higher organisms such as plants are far more complex in their genome and metabolic pathways. This makes them significantly more challenging for synthetic biologists work with. Successfully re-engineering the metabolic pathways of microbes has provided excellent training toward the ultimate goal of using plants as hosts. By collaborating with CSRS researchers specializing in plant science, the Cell Factory Research Team is translating its pioneering work in microbes into insights that can accelerate plant cell synthetic biology, particularly for the production of the terpenoids used in medicines and aromatics.
With the Japanese government recently announcing its aim to be carbon neutral by 2050, higher plants that can fix carbon dioxide using the energy from sunlight is the absolute ideal for future chemical production.
References
- 1. Noda, S., Shirai, T., Mori, Y., Oyama, S. & Kondo, A. Engineering a synthetic pathway for maleate in Escherichia coli. Nature Communications 8, 1153 (2017) . doi: 10.1038/s41467-017-01233-9
- 2. Mori, Y., Noda, S., Shirai, T. & Kondo, A. Direct 1,3-butadiene biosynthesis in Escherichia coli via a tailored ferulic acid decarboxylase mutant. Nature Communications 12, 2195 (2021). doi: 10.1038/s41467-021-22504-6
- 3. Imada, M., Takenaka, Y., Hatanaka, H., Tsuge, T., Abe, H. Unique acrylic resins with aromatic side chains by homopolymerization of cinnamic monomers. Communications Chemistry 2, 109 (2019). doi: 10.1038/s41563-021-01122-z
About the Researchers
Kazuki Saito, Director, RIKEN Center for Sustainable Resource Science
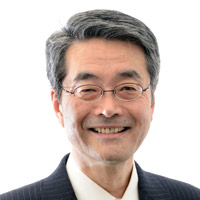
Kazuki Saito obtained his PhD in bio-organic chemistry and biochemistry from the University of Tokyo in 1982. In 1995, he became a professor at Chiba University, where he stayed until retirement in March 2020. He is currently a Professor Emeritus at Chiba University. Since 2005, he has also served as a Group Director at the RIKEN Center for Sustainable Resource Science (CSRS) (formerly, RIKEN Plant Science Center), where he directs metabolomics research. In April 2020, he became the Center Director. He has been a Highly Cited Researcher in the field of Plant & Animal Science since 2014.
Tomokazu Shirai, Senior Scientist, RIKEN Center for Sustainable Resource Science
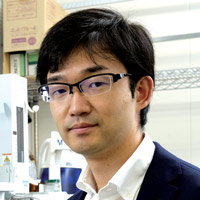
Tomokazu Shirai obtained a PhD in applied biotechnology from Osaka University in 2007. In 2008, he started working at Mitsui Chemicals, Inc., where he was involved in the development of isopropyl alcohol producing E. coli bacteria. In 2012, he joined Cell Factory Research Team, which was part of the RIKEN Biomass Engineering Program and later moved to the RIKEN Center for Sustainable Resource Science (CSRS). Since 2020, he has also been the Deputy Team Leader of the Biomonomer Production Research Team, a project jointly run by RIKEN, the ZEON Corporation and Yokohama Rubber Co. in the RIKEN Baton Zone Program.