Apr. 22, 2024 Perspectives Physics / Astronomy
Building capacity to beat cancer with a targeted radiopharmaceutical
RIKEN’s particle accelerator facilities are producing astatine-211, a radioisotope with great promise for selective anti-tumor therapies, says Hiromitsu Haba.
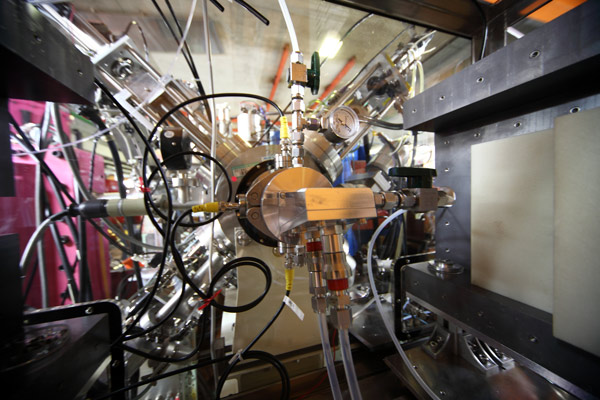
RIKEN has developed a device for the efficient production of astatine-211. The device uses a helium beam that is fired at a bismuth target (found in the aluminum box at the image center). © 2024 RIKEN
The suite of powerful particle accelerators at RIKEN has a long history of dual-purpose use. As well as the central role these instruments play in fundamental nuclear physics research, they have also long been employed in the production of valuable radioisotopes.
Today, some of the strongest radioisotope demand comes from medicine, where they are used in imaging and increasingly, as cancer treatments. Currently, there is a rapidly growing interest in astatine-211, a promising radioisotope for the potential selective treatment of numerous cancers.
Fortunately, innovative new methods for producing astatine-211 in practical amounts have recently been pioneered at our facility. And, a recently launched human clinical trial at Osaka University Hospital of an astatine-211 based anticancer radiopharmaceutical, a first for Japan, is leveraging these new production abilities.
Traditional strengths
Radioisotopes are atoms that have an unstable nucleus, and so shed excess energy as radiation in a process called radioactive decay. These active chemical species have numerous applications across research, industry, and medicine.
At RIKEN, this potential was recognized early. In 1937, one of the world’s first cyclotron particle accelerators was built at RIKEN by renowned physicist Yoshio Nishina. Within a year, Nishina was using the cyclotron to produce valuable radioisotopes to trace the behavior of elements in chemical reactions and plants, in addition to his nuclear physics research. Today our facility, the RIKEN Nishina Center for Accelerator-Based Science, is named in his honor, and the dual function that Nishina introduced remains.
In the research realm, a superheavy chemical element called nihonium—the first element ever discovered in Asia—was first synthesized in our facility in 2004, and our fundamental research into superheavy element synthesis remains active.
On the application side, our radioisotope production expertise has continued to expand. We now produce more than 100 radioisotopes, covering all regions of the periodic table. We collaborate with researchers around the world to conduct radioisotope application studies in the fields of physics, chemistry, biology, engineering, environmental science, and medicine.
In the medical field, there is rapidly increasing interest in astatine-211 as a potential cancer therapy. This radioisotope decays by emitting an alpha particle, which characteristically delivers a large amount of energy over a short distance.
In the human body, an alpha particle shed by astatine-211 will typically travel a distance of no more than two or three cells. Thus, if we can incorporate astatine-211 into a medicine that selectively accumulates in cancer cells, we could create a therapeutic that is highly cytotoxic to cancer cells, but has minimal toxicity to healthy cells. This research could result in new anticancer medicines with high efficacy but few side effects.
Cool operations
In Japan, demand for astatine-211 is increasing rapidly. This isotope does not exist in nature, but it can be produced by particle accelerators. We produce it by firing a beam of helium-4 particles into a target of solid metallic bismuth. Helium has an atomic number of two, and bismuth an atomic number of 83. When the two atomic nuclei combine, they form the element with atomic number 85: astatine.
The key challenge with astatine-211 production is that metallic bismuth has a relatively low melting point, liquifying at just 271°C. The helium-4 particle beam generates a lot of heat and melts the bismuth target. To increase our production rate to meet growing demand, we introduced several process modifications to minimize the heating effect.
We orient the bismuth target at an angle of 10° to the beam axis, and use helium gas and flowing water to cool the front and back of the target, respectively. We also use a beam wobbling system to continually sweep the beam across the target, avoiding local heating of the beam spot on the target.
With this system, we increase the beam power—and astatine-211 production rate—without melting the target. The 25-particle microampere helium-4 beam—one of the world's highest beam currents for astatine-211 production in its class—is used in our Azimuthally Varying Field (AVF) Cyclotron, a spiral-shaped particle accelerator built for use in a variety of research fields, ranging from nuclear physics to medicine.
We have also introduced a fast and efficient astatine-211 purification method that uses dry distillation. After collecting the irradiated target from the accelerator, we can simply heat it and the volatile astatine-211 separates from the remaining bismuth as a gas. We then attach the astatine-211 to a cancer-targeting molecular carrier, creating the radiopharmaceutical.
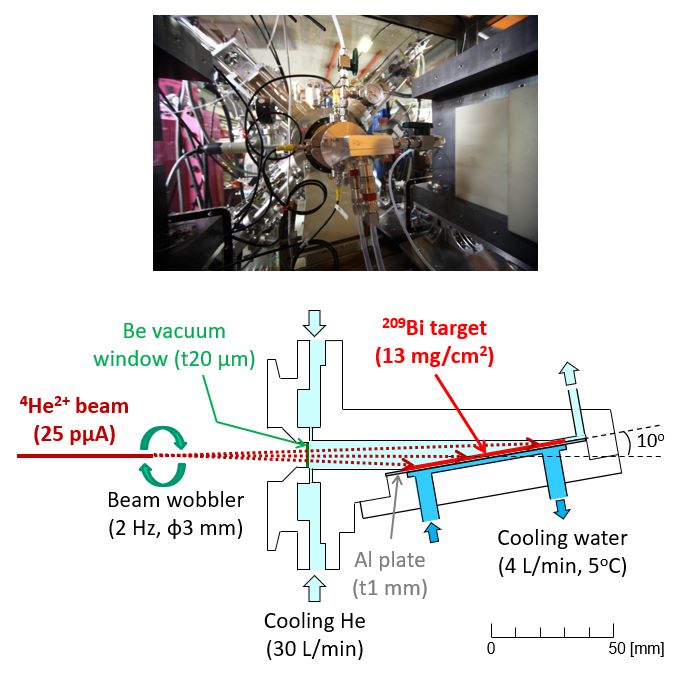
Figure 1. Water- and helium-cooled target irradiation system. The bismuth target is placed at an angle of 10 degrees to the beam axis to increase the cooling area. The beam axis is rotated to suppress melting of the bismuth by the high-intensity beam irradiation. The produced astatine-211 is extracted from the bismuth target using a chemical separation device. © 2024 RIKEN
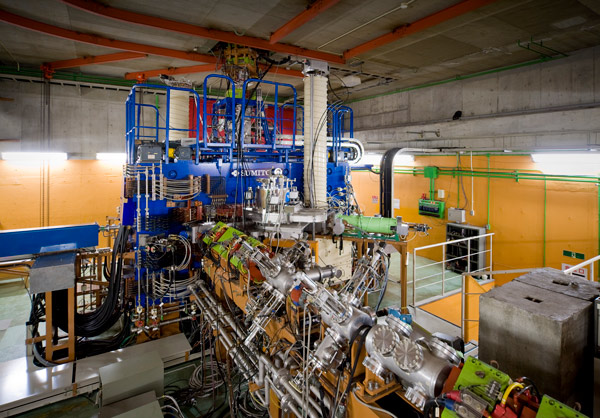
Figure 2: Researchers are making the astatine-211 radioisotope by using particle accelerators such as the RIKEN Azimuthally Varying Field (AVF) Cyclotron to fire a beam of helium-4 particles into a bismuth target. © 2024 RIKEN
Combating cancer
With a half-life of just 7.2 hours, the rapid decay of astatine-211 imposes significant restrictions on transportation time, limiting the delivery area. The larger the batch that we can make, however, the further we can transport it and still have a useful amount left once the sample reaches its destination.
With our improved astatine-211 production technique we can now distribute our product to many researchers in Japan. We currently support approximately 20 research groups with our astatine-211. In one recent highlight, our product was used for Japan’s first clinical trial of the targeted alpha-particle therapy for thyroid cancer at Osaka University Hospital. This was the first time our product has been used for human treatment.
A second experimental astatine-211 radiopharmaceutical, also developed with Osaka University, will be tested in a human clinical trial for another cancer in 2024.
Increasing our astatine-211 production rate also means we can reduce the expense of these cancer drugs. The particle accelerator’s running costs are very high, so if we can increase the production rate by using stronger beams, we can reduce accelerator beam time, which reduces drug development costs and, subsequently, drug prices. In addition, if we can produce more astatine in the same amount of bismuth, we can also increase the purity of the final shipped product, because the total amount of chemical impurities introduced during the chemical process remains the same.
For these reasons, we continue to develop improved methods for making astatine-211. Our latest advance deploys a production system based on a rotating liquid bismuth target. This innovation removes the need to keep the temperature very low, opening the possibility of using even more powerful beams.
Current research
In our liquid bismuth process, developed with the Metal Technology Company, a business based in Tokyo that produces advanced metal processing technology, the bismuth is placed in a tilted, rotating cup. As the cup is heated to 300–400°C, and spun at 2,000 revolutions per minute, the molten bismuth spreads to coat the wall of the cup due to centrifugal force. The liquid bismuth is then targeted with a powerful helium-4 beam.
In a further efficiency boost, the system has been designed so that we can collect the astatine-211 easily from the accelerator. As the astatine-211 turns onto a gas from the hot bismuth target, it is swept from the accelerator in a stream of helium carrier gas, passing via tubing into an adjacent lab where it is captured using a trap cooled to −196°C. So, the astatine-211 can be collected in a continual process as the molten bismuth is irradiated, or in a separate step post-irradiation.
We have currently installed this liquid bismuth system on one of RIKEN’s powerful accelerators, called the RIKEN Ring Cyclotron, which is operating with a beam power of 25 particle microampere or more. In April 2024, however, we are planning to install the system on our most powerful instrument, the recently upgraded superconducting RIKEN linear accelerator (SRILAC).
As with RIKEN’s original 1930s cyclotron accelerator, this upgrade was initially planned with fundamental research in mind. In its prior form, this linear accelerator was used in our synthesis of nihonium, one of our research center’s greatest discoveries. To access even heavier new elements, we have upgraded this instrument by installing a new superconducting ion beam source and accelerator to increase the beam current and energy. Our target is to synthesize a new superheavy element, element-119, which has never previously been made.
With its very powerful particle beams, however, this accelerator should also be invaluable for the large-scale production of astatine-211. We are currently commissioning a new beamline on this apparatus for astatine-211 production based on our rotating cup technology, gradually increasing the beam current to until we reach about 100 particle microamperes.
With this advance, we will continue the strong dual tradition of developing accelerators for both practical and fundamental research at the RIKEN Nishina Center for Accelerator-Based Science.
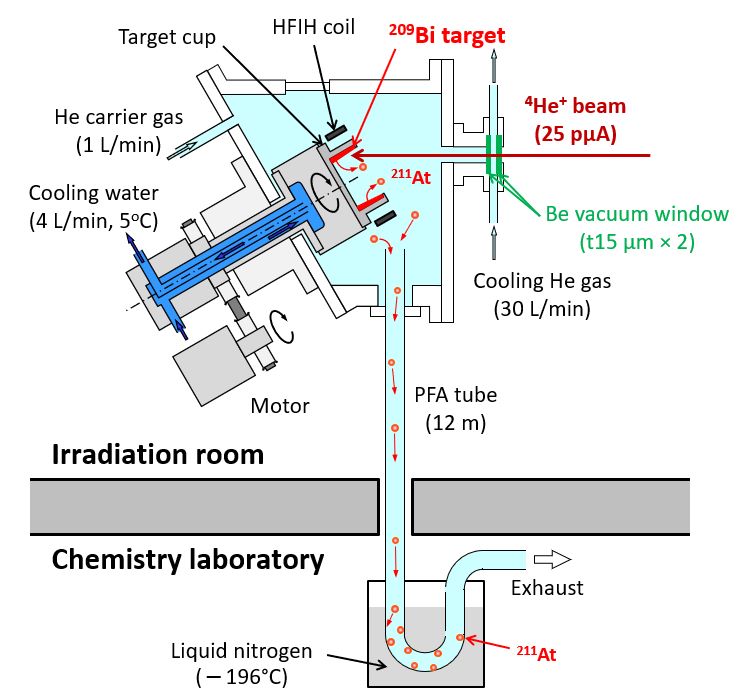
Figure 3: Schematic of the newly developed astatine-211 production system with the Metal Technology Company. By rotating the target cup, a centrifugal force maintains the target shape even if the target melts due to the irradiation. The cup is heated with a high-frequency induction heater (HFIH) and astatine-211 sublimated from the target is transported to a chemistry laboratory by helium gas stream. Thus, chemical separation of asttain-211 can be done immediately after or during irradiation to reduce its radioactive decay loss. © 2024 RIKEN
Rate this article
Related links
- 1.A new generic treatment for multiple types of cancer
- 2. T. Watabe, et al. Targeted α-therapy using astatine (211At)-labeled PSMA1,5,and 6: a preclinical evaluation as a novel compound. European Journal of Nuclear Medicine and Molecular Imaging 50, 849–858, (2023). doi: 10.1007/s00259-022-06016-z
About the researcher
Hiromitsu Haba, Director, Nuclear Chemistry Group, RIKEN Nishina Center for Accelerator-Based Science
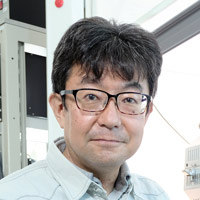
Hiromitsu Haba received his Ph.D. from Kanazawa University in Japan in 1999. Next he worked at the Japan Atomic Energy Institute as a postdoctoral researcher, and then at RIKEN as a special postdoctoral researcher, research scientist, senior research scientist, and team leader. In 2018, he became director of the Radioisotope Application Research Group, then director of the Superheavy Element Research Group and later director of the Nuclear Chemistry Group at the RIKEN Nishina Center for Accelerator-Based Science. He is also a visiting professor at the Graduate School of Science and Technology at Niigata University, Japan. His scientific interests focus on nuclear and radiochemistry, with a particular speciality in the synthesis and chemistry of superheavy elements and the production and application of radioisotopes made using accelerators.