Feb. 2, 2007 Research Highlight Physics / Astronomy
Antimatter cools off
Chilled antihydrogen could reveal wrinkles in nature’s symmetry
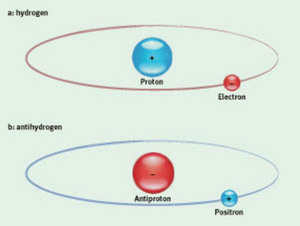
Physicists have proposed a novel way to cool antimatter, which should make it much easier to study in detail. Trapping useful amounts of the substance may be the key to working out why matter dominates antimatter in making up the fabric of the universe. However, no efficient method of cooling and storing antimatter has yet been developed, limiting these experimental investigations.
Most of the atoms in existence are hydrogen, made of a positive proton circled by a negative electron. Yet in 1932, physicists discovered that the electron had a mirror particle with exactly the same mass, but carrying a positive charge. They called it the positron (Fig. 1). As more of these antiparticles were found, it became clear that every particle in nature has a symmetrical antiparticle—an evil twin. If the two collide, they annihilate each other in a burst of energy.
In the early 1990s, scientists created the first anti-atoms by combining positrons with negative antiprotons to make antihydrogen (Fig. 2). However, this recipe produces hot anti-atoms that are unsuitable for sensitive measurements.
Scientists want to trap antihydrogen in a way that allows them to cool it down, so that they can compare it with hydrogen. Although the standard theory predicts that matter and antimatter are symmetrical, more sensitive tests might show some slight asymmetry. This would not only help to refine our understanding of nature, it may also answer the puzzle about the imbalance of antimatter and matter in our universe. Hot antihydrogen is simply no use for these studies, explains Yasunori Yamazaki, a physicist at the RIKEN Discovery Research Institute in Wako. Instead, the anti-atoms must be cooled almost to absolute zero (-273 ˚C), the coldest temperature possible.
Too precious for evaporative cooling
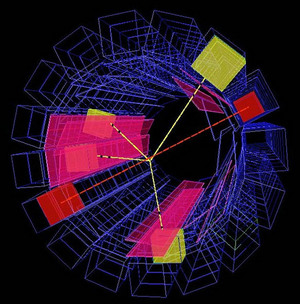
Hydrogen atoms can be chilled to these extremes relatively easily if they are contained within magnetic fields that act like a well. Changing the shape of this well by varying the magnetic fields allows the hottest atoms to spill over the sides of the trap, just like boiling water spurting out of the spout of a kettle. As the hot hydrogen atoms leave, they carry energy with them and leave cooler atoms behind. Subsequent collisions between the atoms remaining in the trap makes sure that there are always a few more ‘hottest’ atoms waiting to leak out over the lip of the trap.
But this process uses up trillions of hydrogen atoms, which are lost during the evaporative cooling process. That’s no problem if you are trying to cool the most abundant element in the universe—but antihydrogen, on the other hand, is incredibly rare. “The total number of antihydrogen atoms ever synthesized may be around one million,” estimates Yamazaki. Researchers simply cannot afford to lose most of their precious antihydrogen by evaporation. “It is practically possible to use evaporative cooling in the case of hydrogen atoms, but not in the case of antihydrogen atoms,” says Yamazaki.
So he and his colleagues, including his doctoral student Yugo Nagata, at RIKEN, the University of Tokyo, and the Harvard-Smithsonian Center for Astrophysics in Cambridge, US, have come up with an alternative cooling method.
Setting a new trap
When antihydrogen is synthesized it is hot and energetic, with the positron and antiproton relatively far apart—this separation means that the electrical attraction between them dominates any other subtle measurements that might point to an asymmetry. But over time, the hot anti-atoms will spontaneously lose energy by emitting radiation. During this process, the positrons fall closer towards the antiproton until they achieve their lowest energy level, or ground state. As reported in Physical Review Letters1, Yamazaki and his colleagues have used quantum mechanical calculations to prove that this decay process could be harnessed to cool samples of antimatter, because it provides a way to efficiently eject energy from the trapped antimatter.
They propose using a ‘cusp trap’, similar to those used to contain hydrogen atoms but relying on a magnetic field formed by two solenoids with opposite currents. This will contain positrons, antiprotons and neutral antihydrogen atoms, all in the same spatial region. “In the cusp trap scheme, antiprotons and positrons can be accumulated very stably,” says Yamazaki.
The scientists’ calculations also show that the decay rate of the excited antihydrogen atoms—and hence the efficiency of cooling—gets higher in stronger magnetic fields, which are found at the edges of the trap. As well as efficient cooling, the trap could also store the antimatter effectively. “The lifetime of the antimatter is expected to be very long, something like 10 to 100 seconds or even longer, considering the results of previous experiments with sodium atoms,” explains Yamazaki. That’s important because “one must trap antihydrogen atoms for a while to get them into their ground state,” he adds.
In other calculations, the team has also shown that by carefully controlling the temperature and magnetic fields within the system, it should also be possible to form beams of antihydrogen atoms. Overall, the scientists predict that cooling close to absolute zero should be possible using their technique.
“At the moment, I do not believe that this is difficult,” says Yamazaki. “But still it should be experimentally proved before we say it’s easy.”
References
- 1. Pohl, T., Sadeghpour, H. R., Nagata, Y. & Yamazaki, Y. Cooling by spontaneous decay of highly excited antihydrogen atoms in magnetic traps. Physical Review Letters 97, 213001 (2006). doi: 10.1103/PhysRevLett.97.213001
About the Researcher
Yasunori Yamazaki and Yugo Nagata
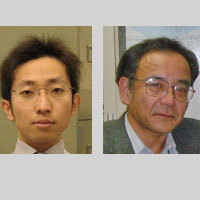
Yasunori Yamazaki was born in Osaka, Japan, in 1949. He graduated from the Department of Physics, Osaka University, in 1973, received his master’s degree in 1975, and in 1978 received his doctoral degree from the Department of Applied Physics at the same university. He was appointed as a research associate of the Tokyo Institute of Technology in 1978, an associate professor of the University of Tokyo in 1988, and a professor at the same university in 1993. He was also appointed as a chief scientist at RIKEN in 1997. His research subjects are the application of beam physics to various fields of natural science, such as biology, as well as the study of antimatter.
Yugo Nagata was born in Matsuyama, Japan, in 1980. He graduated from the Department of Natural and Environmental Science, Tokyo Gakugei University, in 2002, and is now studying for his doctoral degree at the Department of Basic Science, University of Tokyo. He has been the Junior Research Associate at the Atomic Physics Laboratory, RIKEN since 2006. His research interests are dynamic collision processes involving antimatter and strong fields.