Mar. 28, 2008 Research Highlight Biology Physics / Astronomy
Synchronized spiking
Oscillating neurons knock their neighbors into step to produce signals that can survive the journey through the nervous system
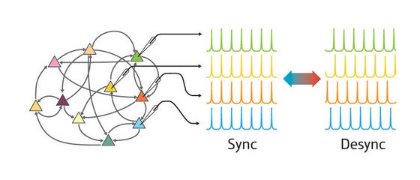
Many natural and artificial systems are made up of oscillators that have to be synchronized. Tomoki Fukai and co-workers at the RIKEN Brain Science Institute in Wako have described how oscillating neurons in the brain work together to produce strong electrical signals1.
“In response to a constant stimulus, neurons typically generate near-periodic action potentials or spikes,” says Fukai. “In the brain, rhythmic neuronal activity is often linked to cognitive behavior. These periodic spiking neurons can be regarded as oscillators.”
Neurons relay information to other neurons via the synapses that connect up the nervous system. A spike from a single neuron may not be strong enough to activate a neuron further down the line, because the signal power is dissipated in the synapses. Therefore it is important that the periodic spikes from several neighboring neurons can be synchronized into one stronger signal (Fig. 1).
For example, synchronization in the frequency range 30–70 Hz is believed to be crucial for paying attention, while the range 3–8 Hz may play a role in learning and memory. “However these issues are still in debate,” says Fukai.
The behavior of each neuron is dominated by its phase response curve (PRC)—which determines how its output spike is affected by the phase of the input spike. A previous theory called the Kuramoto model has shown how these phase shifts can lead to synchronized states, but it assumes that the PRCs of neurons are all fairly similar. In reality PRCs vary considerably from neuron to neuron, especially across different layers in the cerebral cortex—a point noted by the RIKEN team2.
“In our [new] model, we extend the Kuramoto model to the case where the…phase response curves are heterogeneous in an oscillator network,” says Yasuhiro Tsubo, a member of the team. “Our method is important since in the real world no system is exactly homogeneous.”
Two types of neurons are known: Type-I, in which the phase of oscillation can only be advanced, and Type-II, in which the phase can be advanced or delayed by a signal. This extra flexibility means that Type-II neurons are more likely to become synchronized. The researchers predicted three possible states for the heterogeneous system: perfect synchronization, partial synchronization or perfect desynchronization.
The new model successfully revealed the novel dynamical states in neuron data from the cerebral cortex of rats. It could even be applied to diverse oscillator systems such as semiconductor devices, heartbeats, laser arrays or the luminescence of fireflies.
References
- 1. Tsubo, Y., Teramae, J. & Fukai, T. Synchronization of excitatory neurons with strongly heterogeneous phase responses. Physical Review Letters 99, 228101 (2007). doi: 10.1103/PhysRevLett.99.228101
- 2. Tsubo, Y., Takada, M., Reyes, A.D. & Fukai, T. Layer and frequency dependences of phase response properties of pyramidal neurons in rat motor cortex. European Journal of Neuroscience 25, 3429–3441 (2007). doi: 10.1111/j.1460-9568.2007.05579.x