Dec. 6, 2013 Research Highlight Physics / Astronomy
Conjuring up calcium’s inner magic
The discovery of an exotic calcium isotope with a new ‘magic number’ of nuclear components brings physicists another step closer to a more complete understanding of the fundamentals of atomic nuclei
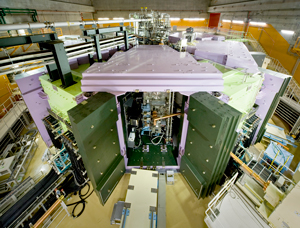
Every naturally occurring heavy element on Earth has its origins in the stars of the Universe. Through a process known as nucleosynthesis, nuclear collisions inside massive celestial bodies fuse together lightweight atoms such as hydrogen and helium to produce heavier and heavier elements, such as carbon and silicon. The natural abundance of elements and their isotopic variants—atoms with an unbalanced number of protons and neutrons—is determined by the stability of the nucleus, which governs whether an astrophysical isotope will be long-lived or susceptible to rapid radioactive decay.
One way that physicists gauge the stability of atomic nuclei is with the concept of ‘magic numbers.’ Similar to the way that electrons travel in tightly defined ‘shells’ around a central nucleus, protons and neutrons also occupy distinct orbits inside the nucleus. When the number of protons or neutrons completely fills a particular shell, corresponding to 2, 8, 20, 28, 50, 82 or 126 particles (the magic numbers), the isotope gains exceptional stability. Such nuclei take on a spherical shape and are difficult to excite due to a significant energy gap that must be overcome before the nucleus can accept new protons and neutrons.
Now, a research team led by David Steppenbeck from the University of Tokyo and Hiroyoshi Sakurai and Satoshi Takeuchi from the RIKEN Nishina Center for Accelerator-Based Science has uncovered evidence of a new magic number of 34 neutrons in the calcium-54 (54Ca) isotope1. The finding is significant because the isotope displays surprising stability despite its seemingly volatile nuclear structure.
Exotic evolution
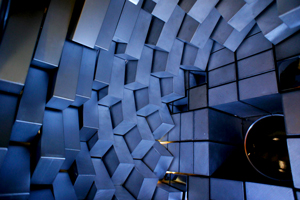
When the theory of magic numbers was developed over a half-century ago, researchers believed that nuclear shells were robust and constant. These notions began to change, however, with studies into short-lived exotic nuclei that have quite different properties from those of natural isotopes, which have balanced numbers of protons and neutrons. In exotic nuclei with significantly more neutrons than protons, the excess neutrons begin to fill normally empty orbital shells, producing extra forces not seen in stable isotopes. “These attractive and repulsive forces cause the orbitals to shuffle about,” explains Steppenbeck. “In some cases, the large energy gaps associated with the magic numbers of standard stable nuclei can disappear and new energy gaps—the onset of new magic numbers—can materialize.”
In order to better understand how changes in shell structure—or ‘shell evolution’—occur, researchers are increasingly turning to calcium isotopes. This element has a magic number of 20 protons, and evidence has emerged that an exotic calcium isotope with 32 neutrons (52Ca) has a substantial energy gap and therefore forms a relatively inert nuclear core. Theoretical calculations have also suggested that 54Ca, which has 20 protons and 34 neutrons, should show magic-number stability, while other studies have cast doubt on this proposition.
“Prior to our experiment, we simply did not know whether the magic number of 34 neutrons would exist in calcium or not,” says Steppenbeck. “There are a great number of theories that predict different characteristics of the 54Ca excited states. Some predict a shell closure with 34 neutrons that is just as large as the traditional one seen with 28 neutrons, while others predict no shell closure at all. Clarifying which theories are correct was a major motivation behind our work.”
Going for a knockout
Resolving the uncertainty surrounding 54Ca isotopes is inherently challenging because the substance is extremely difficult to produce and study in the laboratory. Fortunately, the team had access to a facility with far from ordinary capabilities: the Radioactive Isotope Beam Factory (RIBF), jointly operated by RIKEN and the University of Tokyo. The superconducting ring cyclotron (Fig. 1) and powerful electromagnets at the RIBF allow researchers to generate beams of specific heavy isotopes travelling at around 60 percent of the speed of light. “No other facility in the world can currently match the high-intensity radioactive ion beams available at the RIBF,” notes Steppenbeck.
In their experiment, the team bombarded a beryllium plate with the isotopes scandium-55 (55Sc) and titanium-56 (56Ti), both of which have 34 neutrons but do not display magic-number stability. Occasionally, these collisions can knock out a single proton from 55Sc, or two protons from 56Ti, to form the elusive 54Ca isotope. By applying careful separation and purification techniques, the researchers produced a beam of 54Ca and proceeded to study its characteristics in detail.
To determine if 34 was indeed a magic number, Steppenbeck and his colleagues excited 54Ca to its higher-energy excited state and watched it decay back to its original state. During the decay process, the isotope emitted gamma rays that the team tracked with a high-efficiency detector array (Fig. 2). The gamma radiation signatures revealed a large energy gap between the ground state of 54Ca and its next shell level—clear evidence of a new magic number. Furthermore, the researchers note that 54Ca is ‘doubly magic’ because both of its proton and neutron shells are filled.
A three-body solution
This discovery promises to give physicists important insights into predictions of unstable nuclei. Investigating how calcium isotopes contribute to element-forming reactions, however, requires theoretical calculations that are currently at the frontier of computational capabilities. Steppenbeck notes that a key finding of the study is that complex ‘three-body’ forces between triplets of subatomic particles should be incorporated into the toolkits of theoreticians to enable experimental work to be reproduced with greater accuracy. “Understanding the properties of neutron-rich nuclei and the forces between protons and neutrons could lead to a better understanding of astrophysical processes.”
References
- 1. Steppenbeck, D., Takeuchi, S., Aoi, N., Doornenbal, P., Matsushita, M., Wang, H., Baba, H., Fukuda, N., Go, S., Honma, M. et al. Evidence for a new nuclear ‘magic number’ from the level structure of54Ca. Nature 502, 207–210 (2013). doi: 10.1038/nature12522
About the Researcher
David Steppenbeck
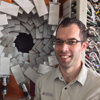
David Steppenbeck was born in the United Kingdom in 1983. He graduated from the University of Manchester in 2005 and obtained his PhD in experimental nuclear physics in 2009 from the same university. Following this, he moved to Japan to join the Radioactive Isotope Physics Laboratory at the RIKEN Nishina Center for Accelerator-Based Science (RNC) under RIKEN’s Foreign Postdoctoral Researcher program. In 2012, he moved to the Center for Nuclear Study at the University of Tokyo where he currently works as a postdoctoral researcher. His research explores the structures of unstable nuclei using radioactive beams provided by the Radioactive Isotope Beam Factory at the RNC.