Dec. 28, 2018 Feature Highlight Physics / Astronomy
Rapid cooling reveals superpowers
Rapid electrical cooling bypasses other states and turns on a superconducting state in materials that were previously not superconductors
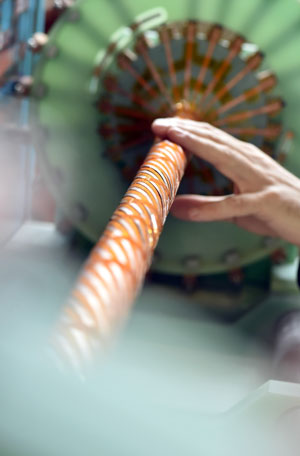
RIKEN researchers have developed a brand-new method for inducing superconductivity, by very rapidly cooling a target material using electrical pulses and effectively bypassing other states that might be more stable. RIKEN’s Hiroshi Oike says it will vastly broaden the range of known superconducting materials.
In most conductors, the natural kinetic motion of warm atoms somewhat disrupts the flow of electrons (electricity), some of which are lost as heat. However, when a superconductor’s atoms are very cold, they stop vibrating around as vigorously, and electrons are free to zoom along the material without resistance because of quantum mechanical effects. Because of this, superconductors don’t suffer from energy scattering and loss, and they can harness larger electric currents.
What Oike and his colleagues from the RIKEN Center for Emergent Matter Science and the University of Tokyo realized is that electron ordering takes time, so reducing the temperature of a potential superconductor extremely fast could circumvent other non-superconducting states that might otherwise take precedence. The group describe it as a “thermal quenching approach” based on the fact that within a time range, a system may be trapped in a local minimum state. In an overview on Oike’s study, Nature compared the idea to plunging hot steel into cold water to harden it, which bypasses small changes that happen during drawn out cooling.
Excitingly, the group found that, in the right conditions, superconductivity does indeed emerge as ‘a metastable state’—the state with the longest lifetime against other excited states—even if more stable competing orders were bypassed during cooling.
Important implications exist if new superconductors are found that operate at higher temperatures than those we already know. Currently, superconducting materials only exhibit this state at very low temperatures—the highest temperate a superconductor is known to operate at is −70°C. Finding higher temperature superconductors could vastly reduce the need for cumbersome cooling apparatus. This change could quickly dovetail into more efficient computing and electrical devices, and MRI machines would be able to finally shed their distinct bulky doughnut shape, which is created by huge liquid cooling systems surrounding superconductors emitting the powerful magnetic fields essential to an MRI’s function.
An energetic balancing act
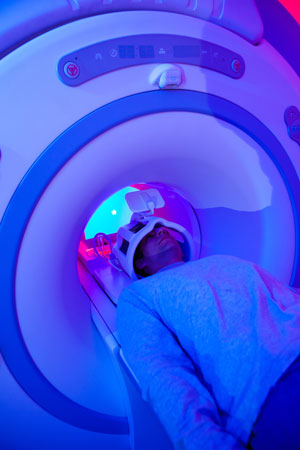
Since the discovery of superconductivity in 1911, scientists have tweaked superconductors by making small changes to their composition or increasing physical/chemical pressure, both as a means of ensuring that the superconducting state is the lowest energy state. They do this because it’s fundamental to thermodynamics that a system will eventually settle into the state with the lowest energy. According to Oike, the problem for many potential superconductors previously had been the fine energetic balance between a superconducting state and its alternatives.
He explains that localized particles have greater kinetic energy, but smaller repulsion energy, than delocalized ones, and so localized and delocalized states are in very close energetic competition. “Electrons at low temperatures are often localized to minimize the energy cost of their mutual repulsion,” Oike says. “But electrons in a superconducting state are delocalized and consequently pay a larger energetic cost because a delocalized electron has more chance to get close to, and repel, other electrons.” As a result, for many materials another state takes precedence over a superconducting state.
Quick cooling very effectively bypasses other states
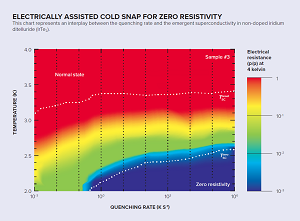
To prove experimentally that their quick cooling idea could effectively line-jump other states, Oike’s team selected a non-superconducting material called iridium ditelluride. The electrons in iridium telluride are delocalized at high temperatures, but usually form an ordered state when slowly cooled. It’s also known from previous research that this material has a superconducting state ‘nearby’ its natural state, which makes it the perfect choice for this experiment.
To cool their iridium ditelluride, the team placed the sample on a cold substrate and then passed rapid pulses of electrical current through it. This, in effect, temporarily turns the sample into an electrical heater, and, every time the current is switched off, the surroundings get warmer but the iridium ditelluride gets much colder.
“In a conventional heater in air, the element cools down by several degrees per minute when it is switched off,” says Oike. “But when the size of the heater is very small and it is attached to a cold substrate, the cooling rate can exceed 10,000,000 degrees per second.” In less than 10 microseconds the pulses heated the metal to more than 27°C before cooling it to −269°C.
The RIKEN team confirmed the creation of a superconducting state by measuring a zero electrical-resistance in their sample at its coolest, and they observed that this non-resistant state remained intact for a week.
Potential superconducting circuits
“I think that the most exciting message from our work is that a non-superconducting material such as iridium ditelluride has the potential to exhibit reversible and non-volatile switching to a superconducting state,” says Oike. “Our scheme means that materials that have been categorized as non-superconducting now have a fresh chance to yield metastable superconducting behavior.”
Achieving this switching phenomenon electrically means that it could also potentially be applied to devices such as superconducting circuits, which are seen as one possible route to quantum computers. And the team is not stopping here: they next hope to investigate other ways of achieving this approach to creating a superconductor.
“Although we used electric current in this study, the temperature of materials can also be controlled with high-intensity light,” says Oike. “When we control the local temperature of a non-superconducting material with focused light, we expect that the illuminated area turns to a superconducting state, thus affording us the ability to write a superconducting area in a non-superconducting material.”
References
- 1. Oike, H., Kamitani, M., Tokura., Y. & Kagawa, F. Kinetic approach to superconductivity hidden behind a competing order. Science Advances 4, eaau3489 (2018) doi: 10.1126/sciadv.aau3489
About the Researcher
Hiroshi Oike
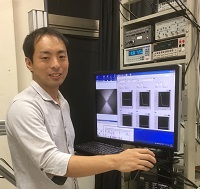
Hiroshi Oike received his PhD from the Department of Applied Physics at the University of Tokyo, while supported by a Research Fellowship for Young Scientists from the Japan Society for the Promotion of Science (DC2). After working at the University of Tokyo as a postdoctoral researcher, he joined the Dynamic Emergent Phenomena Research Unit at the RIKEN Center for Emergent Matter Science in 2013. In 2018, he became a research associate in the Department of Applied Physics at the University of Tokyo. His research interests include phase control of correlated electrons based on the understanding of phase transitions, such as metal–insulator transitions, superconducting transitions, and magnetic transitions.